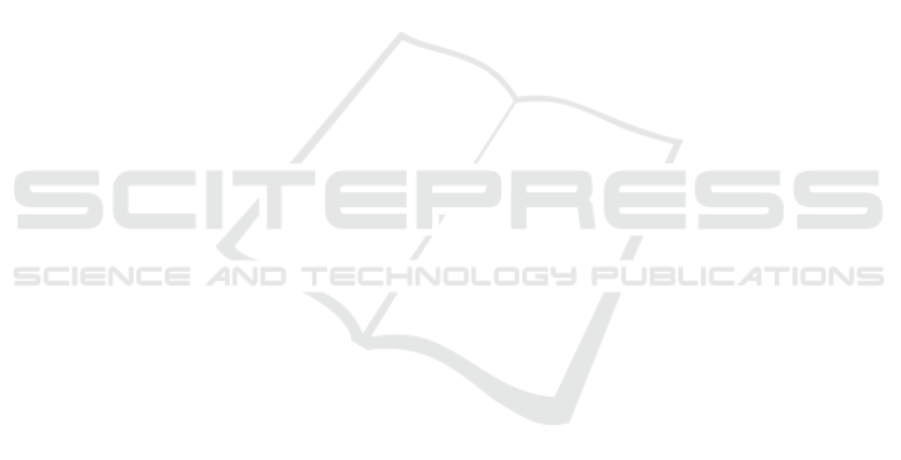
Considering the two paths separately, in the El-
lipse case, cybersickness seems worst, probably
because in this simulations sea was more rough.
So sickness depends on a combine action of game
settings (calm or rough sea), hardware setup (im-
mersive or non-immersive) and speed of the boat.
In other words, the use of VR itself does not ex-
plain the increase of user malaise state.
- Sense of Presence. Sense of presence rates are
higher for VR simulations. Moreover, the trials
with the fast ship have a lower Spatial Presence
but a higher Experienced Realism with respect to
trials with the slow vessel. This is probably due to
the better realism and response speed of the fast
ship. Furthermore, there is a statistically signifi-
cant difference between the Presence Factor in im-
mersive and non-immersive simulations. All these
results demonstrate that the use of VR systems al-
lows user to feel more involved and present in the
virtual scenario.
Head rotation angles represent an objective me-
asurement of the degree of the interaction of the
user with the virtual scenario. This evaluation,
though, is subjective, in fact people can be more
or less prone to explore the virtual world surroun-
ding them. Considering each participant separa-
tely, we can notice that head rotations are actually
more limited in the monitor case, scattered around
a central value, which is the initial head rotation
when the application starts. While in the HMD
case, in general, people have a greater propensity
to turn their head, especially in the Eight path with
the fast boat, probably because the higher diffi-
culty of the task leads them to collect more in-
formation from the environment, for example the
development of the path curvature.
In conclusion, this preliminary work highlighted
that the two setups have been proven to be equiva-
lent: in terms of performances there are no differen-
ces and while monitor simulation system causes less
cybersickness, VR setup allows a better sense of pre-
sence. Further acquisitions, on non expert drivers or
senior drivers, are required in order to better under-
stand the usability of the system on a large scale and
its actual usefulness in providing long term learning
of new skills.
ACKNOWLEDGEMENTS
This work was partially funded by the Project “MIT
(Ministero dei Trasporti - Italian Ministry of Trans-
port) - Leadership tecnologica”
REFERENCES
Aldaba, C. N., White, P. J., Byagowi, A., and Moussavi, Z.
(2017). Virtual reality body motion induced navigati-
onal controllers and their effects on simulator sickness
and pathfinding. In Engineering in Medicine and Bio-
logy Society (EMBC), 2017 39th Annual International
Conference of the IEEE, pages 4175–4178. IEEE.
Benedict, K., Kirchhoff, M., Gluch, M., Fischer, S., Schaub,
M., Baldauf, M., and Klaes, S. (2014). Simula-
tion augmented manoeuvring design and monitoring-
a new method for advanced ship handling. TransNav,
International Journal on Marine Navigation and Sa-
fety od Sea Transportation, 8(1).
Bos, J. E., de Vries, S. C., van Emmerik, M. L., and Groen,
E. L. (2010). The effect of internal and external fields
of view on visually induced motion sickness. Applied
ergonomics, 41(4):516–521.
Bouchard, S., Robillard, G., Renaud, P., and Bernier, F.
(2011). Exploring new dimensions in the assessment
of virtual reality induced side effects. Journal of com-
puter and information technology, 1(3):20–32.
Coxon, M., Kelly, N., and Page, S. (2016). Individual dif-
ferences in virtual reality: Are spatial presence and
spatial ability linked? Virtual Reality, 20(4):203–212.
Davis, S., Nesbitt, K., and Nalivaiko, E. (2014). A syste-
matic review of cybersickness. In Proceedings of the
2014 Conference on Interactive Entertainment, pages
1–9. ACM.
Davis, S., Nesbitt, K., and Nalivaiko, E. (2015). Comparing
the onset of cybersickness using the Oculus Rift and
two virtual roller coasters. In Proceedings of the 11th
Australasian Conference on Interactive Entertainment
(IE 2015), volume 27, page 30.
Gianaros, P. J., Muth, E. R., Mordkoff, J. T., Levine,
M. E., and Stern, R. M. (2001). A questionnaire for
the assessment of the multiple dimensions of motion
sickness. Aviation, space, and environmental medi-
cine, 72(2):115.
Kennedy, R. S., Lane, N. E., Berbaum, K. S., and Li-
lienthal, M. G. (1993). Simulator sickness question-
naire: An enhanced method for quantifying simulator
sickness. The international journal of aviation psy-
chology, 3(3):203–220.
Keshavarz, B. and Hecht, H. (2011). Validating an efficient
method to quantify motion sickness. Human factors,
53(4):415–426.
Kim, Y. Y., Kim, H. J., Kim, E. N., Ko, H. D., and Kim,
H. T. (2005). Characteristic changes in the physiologi-
cal components of cybersickness. Psychophysiology,
42(5):616–625.
Lawson, B. and Mead, A. (1998). The sopite syndrome
revisited: drowsiness and mood changes during real
or apparent motion. Acta astronautica, 43(3-6):181–
192.
McGill, M., Ng, A., and Brewster, S. (2017). I am the
passenger: How visual motion cues can influence
sickness for in-car VR. In Proceedings of the 2017 chi
conference on human factors in computing systems,
pages 5655–5668. ACM.
HUCAPP 2019 - 3rd International Conference on Human Computer Interaction Theory and Applications
72