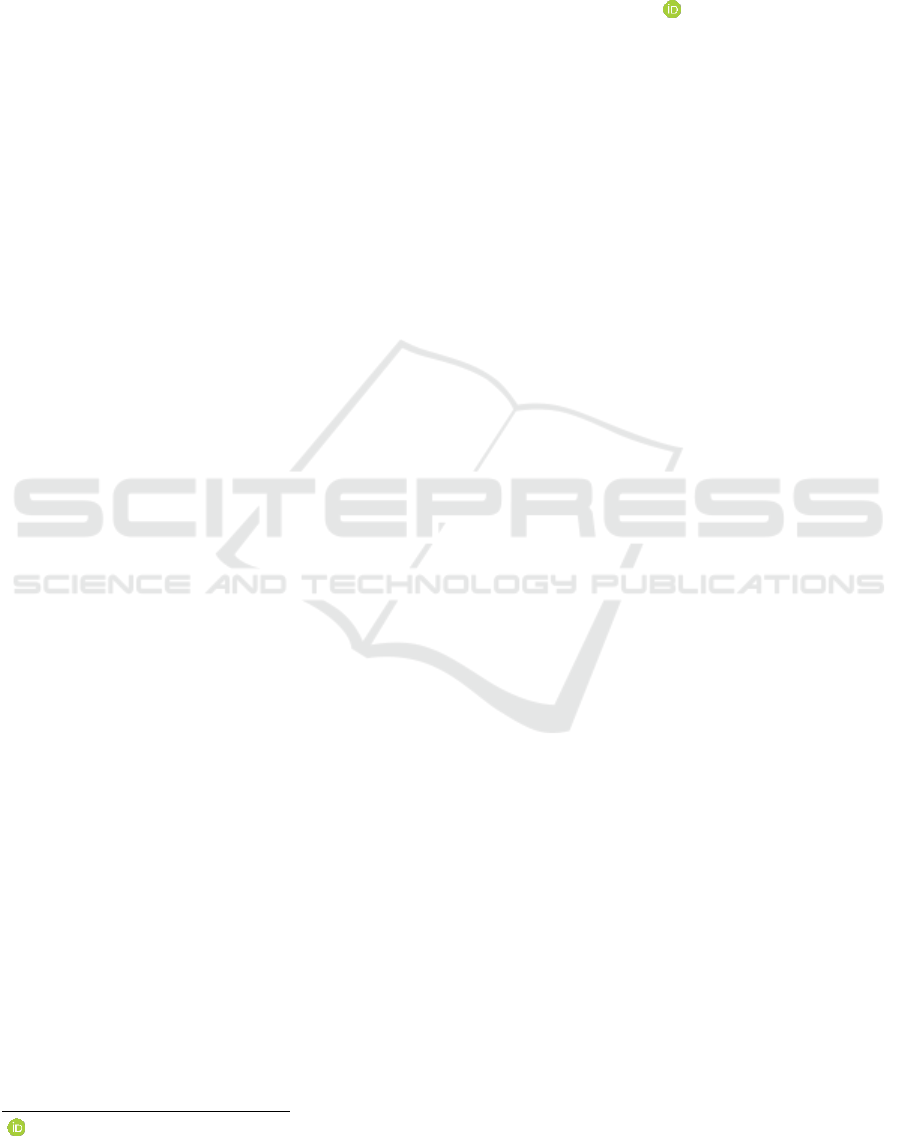
Characterization of the Bias Current Behavior in a SOA for
Linearizing Amplification in a CO-OFDM System
Julio C. Ortiz-Cornejo
1
and Jorge A. Pardiñas-Mir
2 a
1
Technological University of Jalisco, Guadalajara, Mexico
2
Department of Electronics, Systems and Informatics, ITESO University, Tlaquepaque, Mexico
Keywords: CO-OFDM, Semiconductor Optical Amplifier, Bias Current, Linearization, Error Vector Magnitude.
Abstract: This paper presents an analysis of the semiconductor optical amplifier (SOA) on coherent optical orthogonal
frequency division multiplexing system (CO-OFDM), when it is used as a booster power amplifier. The
semiconductor optical amplifier is driven by their bias current injection, which control the amplification level.
In this sense, this study analyses the impact of bias current change on a SOA based CO-OFDM system. It is
well known that SOA is prone to nonlinear distortion when high input power is used at high gain level, because
the gain saturation. In addition to the aforementioned analysis, here it is also presented three operation
scenarios to characterize the performance of the system: maximum EVM equal to 20%, constant output power
equal to -7 dBm, and constant SOA Gain equal to 17 dB. This let us obtain a way to optimize the SOA
performance, under each scenario, controlling the bias current.
1 INTRODUCTION
Amplification process is a crucial step in the coherent
optical orthogonal frequency division multiplexing
(CO-OFDM) systems. Given the inherent nature of
the CO-OFDM signal to generate high peaks of
signal, it condition could drive the optical amplifier to
gain saturation, and signal distortion at the receiver
(Rahmatallah, 2013). Because of it, the optical
amplifiers are one of the key components in the CO-
OFDM systems (Khaleghi, 2013). Recently the
semiconductor optical amplifiers (SOA) has been
extensible studied as a good candidate in CO-OFDM
systems, due to the cost-effective characteristics
(Azou, 2015), (Renaudier, 2019). However, SOA still
have some points to solve, for instance, SOA can
exhibit nonlinear behavior when it operates in
saturation region. These nonlinearities can cause
problems such as frequency chirping and generation
of inter-modulation products (Bendimerad, 2017).
One way to avoid these undesirable effects is to
regulate dynamically the bias current (I
bias
) to control
de SOA amplification, in order to avoid SOA gain
saturation.
The principal application of SOA can be divided
in three main functions: power booster of transmitter,
a
https://orcid.org/0000-0002-0972-3980
in line amplifiers, and optical preamplifier. The
booster amplifiers are placed at the optical transmitter
side to enhance the transmitted power level or to
compensate for the losses of optical elements between
the laser and optical fibers, such as optical couplers,
and external optical modulators. In-line amplifiers are
placed along the transmission link to compensate the
losses incurred during propagation of optical signal.
Optical preamplifiers are used to increase the signal
level before photo-detection occurs, improving the
receiver sensitivity (Shieh, 2010).
This study aims to analyze the impact of bias
current change in the SOA behaivor, used as a booster
for a back-to-back CO-OFDM transmission. To
achieve this goal, we use a simulation system based
on Matlab for digital signal processing of modulation-
demodulation, and ADS for the SOA model
simulation. This simulator has been used before in
(Khaleghi, 2013), (Younes, 2017) showing good
consistence between simulation and experimental
results.
The rest of this report is organized as follows.
Section II presents the simulation results given by the
bias current change on SOA based CO-OFDM system
for a wide range of input power (P
in
) levels. In Section
III we go into the analysis of bias current functions to
Ortiz-Cornejo, J. and Pardiñas-Mir, J.
Characterization of the Bias Current Behavior in a SOA for Linearizing Amplification in a CO-OFDM System.
DOI: 10.5220/0007931502430248
In Proceedings of the 16th International Joint Conference on e-Business and Telecommunications (ICETE 2019), pages 243-248
ISBN: 978-989-758-378-0
Copyright
c
2019 by SCITEPRESS – Science and Technology Publications, Lda. All rights reserved
243