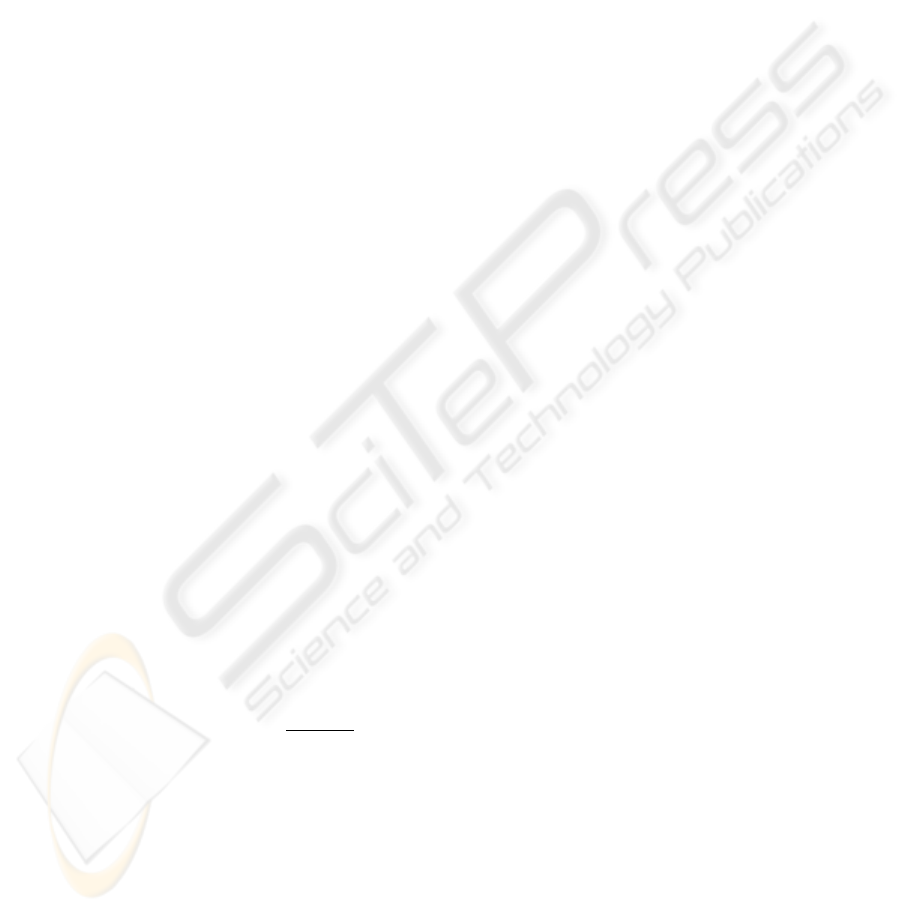
degree of burstiness characterized by a periodic traffic
pattern and a high variance on the rates at which the
data is generated.
The model of HIPERLAN/2 network has been im-
plemented in OPNET 9.0 (OPNET, 1987-2002). We
have measured the jitter among video frames, i.e.,
the time between the last packets of two consecutive
frames and the end-to-end delay for each packet. In
order to limit the delay experienced by the video ap-
plication, an essential condition to guarantee the QoS
required by the video application, the maximum time
that a piece of video data (referred form now on as
packet) can remain in the transmission buffer has been
set to 100 ms. This time limit is on-line with the val-
ues specified in (Karam and Tobagi, 2000).
In order to evaluate the proposed mechanism, re-
ferred from now on as PROP, we carry out a compar-
ative study of our proposed scheme with two schemes
proposed in (Lenzini and Mingozzi, 2001). The first
method therein, referred from now on as MING1 re-
serves a given number of LCH channels and one SCH
channel every given number of frames. In this way,
the MT counts at regular intervals with a given num-
ber of LCH channels for data transmission. The MT
uses the SCH channel to request for more resources
on a timely basis. The second method in (Lenzini and
Mingozzi, 2001), namely MING2, only reserves one
SCH channel every given number of frames. It is clear
that the performance of all three mechanism heavily
relies on parameters, such as the number of reserved
LCH and SCH channels, the frequency at which the
MT´s can place the resource request (RR) messages
and the timer used by the polling mechanism. We start
then by studying the performance of the MING1 and
MING2 schemes by tuning their system parameters
when supporting the video services under consider-
ation. This will set the basis for a fair comparative
study.
Figure 4.(a) shows the end-to-end delay as a func-
tion of the number for the MING1 scheme. We have
varied the number of reserved LCHs per frame as well
as the reservation period of the SCH channel. This
last parameter has been expressed as
#SCH
#frames
. From
the figure, it is clear that the system performance is
severely affected as the number of reserved LCHs is
increased. This is due to the fact that this scheme is
unable to statistically multiplex the traffic. This is par-
ticular important in a system supporting video traffic.
This traffic is characterized by a high variability. This
is clearly shown by the fact that when LCH=4, the
maximum number of MTs is six while for LCH=2,
the maximum number MTs reaches 12. From the fig-
ures, it is also clear that by varying the reservation
period of the SCH does not help when the system op-
erates under heavy load. On the contrary, under low
loads, the periodicity of the SCH has a clear impact on
the system performance. From the figure, a clear ex-
ample is provided for the system consisting of 6 MTs
and making use of 2 LCHs.
Figures 4.(a) and (b) show that the best results are
obtained for the system with the least number of re-
served LCHs and lowest ratio of SCH per frame. It is
important to note that the number of LCHs (LCHs=2)
is given by the average bitrate required by the video
sequence.
In the case of the MING2, no reservation of LCHs
whatsoever is made. However, the AP changes the
rate at which the SCH is assigned to the MTs. Fig-
ure 5.(a) shows the end-to-end delay. This scheme
exhibits similar performance to the one obtained for
the MING1 scheme. At low loads, the performance
on the system improves as the frequency at which the
SCH´s are provided to the MT´s is increased. This is
due to the fact that the MTs can more frequently place
their requests. It is clear that under these load condi-
tions, there are plenty of available resources to fulfill
the MT’s requirements. However at high load condi-
tions, (#MT ≥ 15), the system shows better results as
the SCHs are granted at a lower rate. Once again, this
can be explained to the decrease in the multiplexing
gain due to the resources granted to the connections.
As more resources are reserved, the frequency of the
control slots (to convey the MT’s requests) should be
made less frequent to free resources that can be effec-
tively used for data transmission.
Regarding the jitter, the MING2 exhibits the best
results for the cases when the SCHs are granted at the
lowest rate (longest period), see Figure 5.(b).
In the case of the PROP mechanism, Figure 6.(a)
shows the end-to-end delay. From the figure, it is clear
that the best results are obtained when the timer is set
to 0.04 s. This can be explained by the fact that for
a shorter timer period, more capacity is used to the
SCHs resulting in a reduction of available capacity
dedicated to data transmission (LCHs). In the case
of low loads, the performance is independent of the
value of the timer; since the system is able to promptly
fulfill the MT´s needs. In other words, the system
is able to promptly poll the stations, i.e., before the
expiration of the timer.
Figure 6.(b) shows the jitter for the PROP mecha-
nism. From the figure, it is clear that the best results
are obtained for the case when the timer has been set
to 0.04 s.
In order to make a fair comparison, we have taken
the system configurations exhibiting the best results
for all three schemes. For the case of MING1,
LCH=2, and a SCH every 15 frames. Similarly to
MING1, for MING2, one SCH channel every 15
frames. In the case of PROP, the timer has been set
to 0.04 s.
Figures 7.(a) and 7.(b) show the end-to-end delay
and jitter for all three schemes, respectively. From
A QOS-AWARE RESOURCE REQUEST MECHANISM FOR DELAY SENSITIVE SERVICES OVER TDMA/TDD
WIRELESS NETWORKS
405