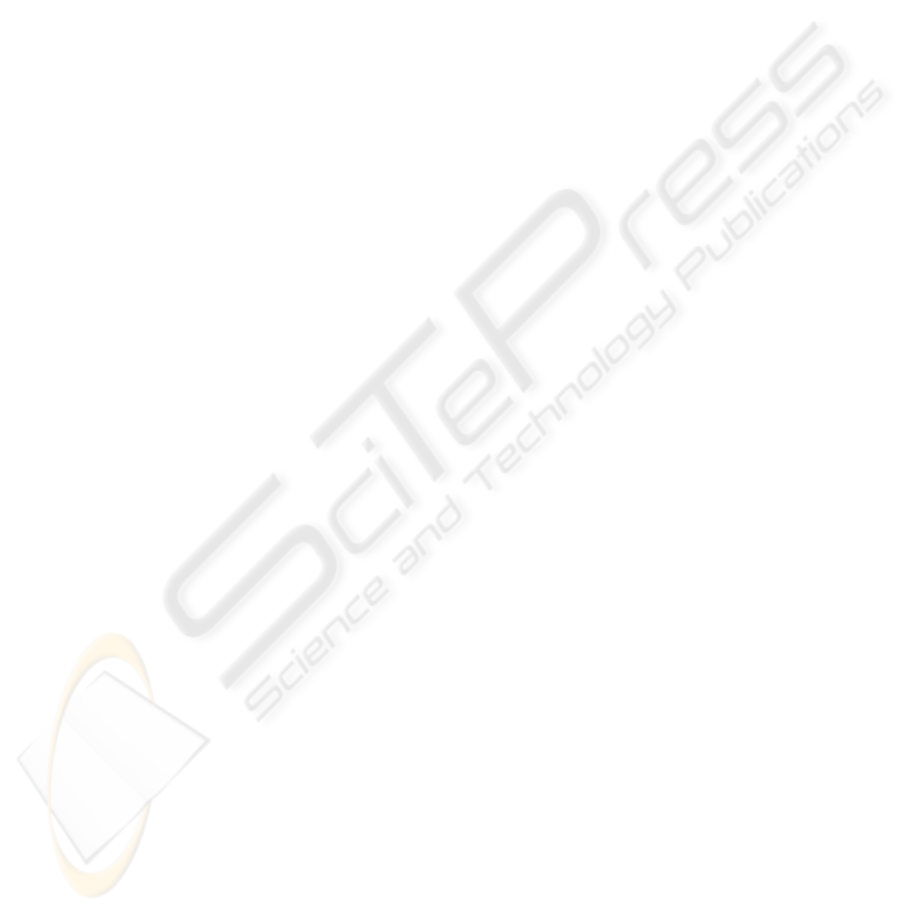
The Origin of Artificial Kinetic
Spectroscopy and Its Application
Ilya Fine
OrSense Ltd., Bergman 2, 76705 Rehovot, Israel
Abstract. Intentionally creating local blood flow cessation at different body sites initi-
ates the time dependent optical response. Specifically, applying circumferential over
systolic pressure at the finger base creates a very stable post-occlusion optical signal
(POS). Geometrically independent features of light absorption and scattering of the
blood are extracted from POS by evaluating the parametric slope (PS). It was shown
that PS, being determined from suitable pairs of wavelengths, correlates with arterial
blood oxygen saturation. Other PS pairs exhibited a strong correlation with blood he-
moglobin. The time dependent signal was simulated in vitro by using the red blood cells
(RBC) aggregation process and was shown to resemble the main features of in vivo
POS
.
1 Introduction
The non-invasive technique for measuring various blood parameters has become very
popular since it eliminates the withdrawal of a blood sample from a patient. Optical
technique utilizes the detection of light response from different locations on the pa-
tient’s body. A commonly used device of this kind, such as the pulse-oximeter, origi-
nates from photoplethysmography (PP). In PP, the light response experiences variable
attenuation due to the pulsation of arterial blood.
Recently, a new approach [1,2], was established, known as Occlusion Spectroscopy,
as an additional method to obtain blood related dynamic spectral characteristics. In
Occlusion Spectroscopy over-systolic pressure is applied to the fleshy tissue to create
a state of temporary blood flows cessation at the measurement site. To optimize this
signal, the PP volumetric affect has to be suppressed.
Once blood flow cessation is established, the optical characteristics start to change
dramatically, changing the detected dynamic signal by about 30%, and sometimes
even by 60%, in comparison to the pulsatile signal that fluctuates only about 2-3%.
The time and spectral kinetic features of the occlusion spectroscopy signal can be
simulated in vitro, by using the RBC aggregation process [5,6].
Our goal was twofold: a) to create a better understanding of POS and b) to elaborate
appropriate algorithmic approaches for utilization in practical applications.
Fine I. (2005).
The Origin of Artificial Kinetic Spectroscopy and Its Application.
In Proceedings of the 1st International Workshop on Biosignal Processing and Classification, pages 110-120
DOI: 10.5220/0001192001100120
Copyright
c
SciTePress