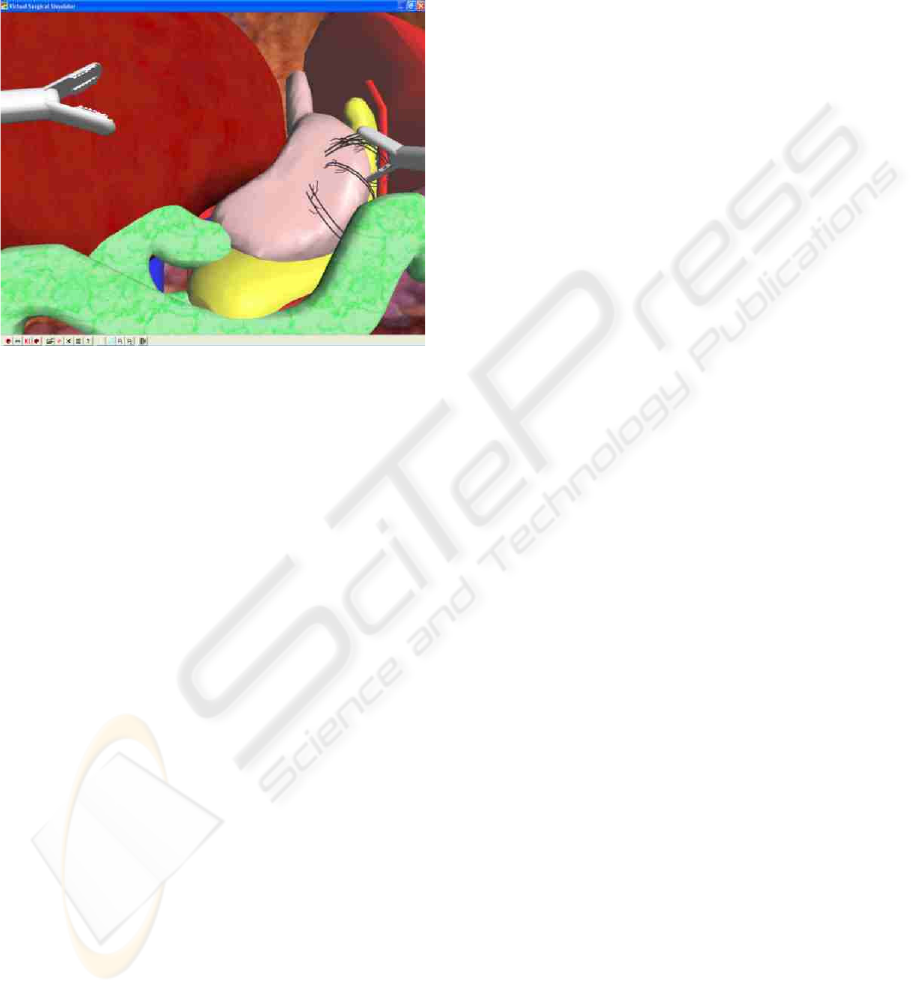
requirements of a VR laparoscopic simulator. If it
was only an issue of graphics, then every surgeon
could have a training and practicing tool on their
laptop PC. It is suitable human interface hardware,
the equivalent of the computer game ‘joystick’ or
‘flight simulator yolk’ that is missing.
Figure 1: A VR laparoscopic surgery application
displaying a representation of the abdomen and two simple
instruments.
When interacting with a 3D virtual environment the
experience not only depends on the type of device
used to provide the human interaction, but also on
external factors such as feedback, whether this is
visual, audio or tactile. Other factors also have to be
considered, such as the number of degrees of
freedom of the user interaction device, as well as
subjective and ergonomic aspects. For example,
(Kontarinis and Howe, 1995) demonstrated that high
frequency vibrations played a significant role in
manipulation tasks. (Lindeman, Sibert and Hahn
1999) showed that adding passive-haptic feedback to
precise manipulation tasks appreciably improved
user performance. (Lindeman, Templeman, Sibert
and Cutler, 2002) demonstrated that adding
vibrotactile feedback to visual and auditory feedback
improved the user experience of virtual
environments.
Force reflecting interfaces such as the
PHANTOM Desktop Device from Sensable
Technologies provide useful feedback, but their
utilization is limited by their cost and the fact that it
is difficult to customize them to fulfill a specific
task.
Vibrating motors, like the ones in mobile
telephones, can be used to provide low-cost
vibrotactile feedback as demonstrated by (Cheng,
Kazman and Robinson, 1996). Indeed vibration
elements have been attached to a standard computer
mouse by (Hughes and Forrest, 1996) to provide
tactile feedback.
This research reflects the belief that integrated
tactile and visual feedback must be implemented in
surgical simulators. The visual feedback is readily
available in real time and at low cost using advanced
computer graphics. The tactile feedback is much
more difficult to integrate into the simulator at low
cost. This paper aims to resolve this issue by
documenting the development of a low cost haptic
device for use in a surgical simulator. Following on
from this introduction, section 2 will outline the
basic design of the mechanical hardware. Sections 3
and 4 describe the electronic system and interface
protocols. The quantum tunnelling sensors are
introduced in section 5 and section 6 illustrates how
the concept was initially tested. Section 7 will then
present conclusions from our work to date.
2 THE SURGICAL TOOLS
This section outlines the design and development of
low-cost haptic devices for interaction with a
surgical simulator.
2.1 The Mk1 Surgical Instruments
The hand grips, and spatial positions used in the
pseudo-instruments, were constructed to match
dimensions taken from actual surgical instruments.
The instruments (the surgical tools) were mounted
on pillars attached to a base. They could rotate from
the horizontal to point down by 45 degrees, and
from facing forward to point inwards by 45 degrees.
These instruments had no electronics attached, as
they were produced solely to verify that the
measurements taken had been translated into an
accurate 3D model. Following initial field trials it
was decided to make some alterations to the position
of the pillars because, during an operation the tool-
tips mostly remain within a 4cm diameter spherical
volume. These changes had the advantage of
allowing more space at the tool-tip end to mount
some electronics in the Mk2 instruments.
2.2 The Mk2 Surgical Instruments
From our earlier research, Mack, Ferguson, Potts
and McMenemy (2006), and discussions with
practicing surgeons it was decided that each joystick
or surgical tool should have six degrees of freedom.
That is, they should allow for movement in the X, Y
and Z axes, rotation about the Z-axis, rotation of the
BIODEVICES 2008 - International Conference on Biomedical Electronics and Devices
30