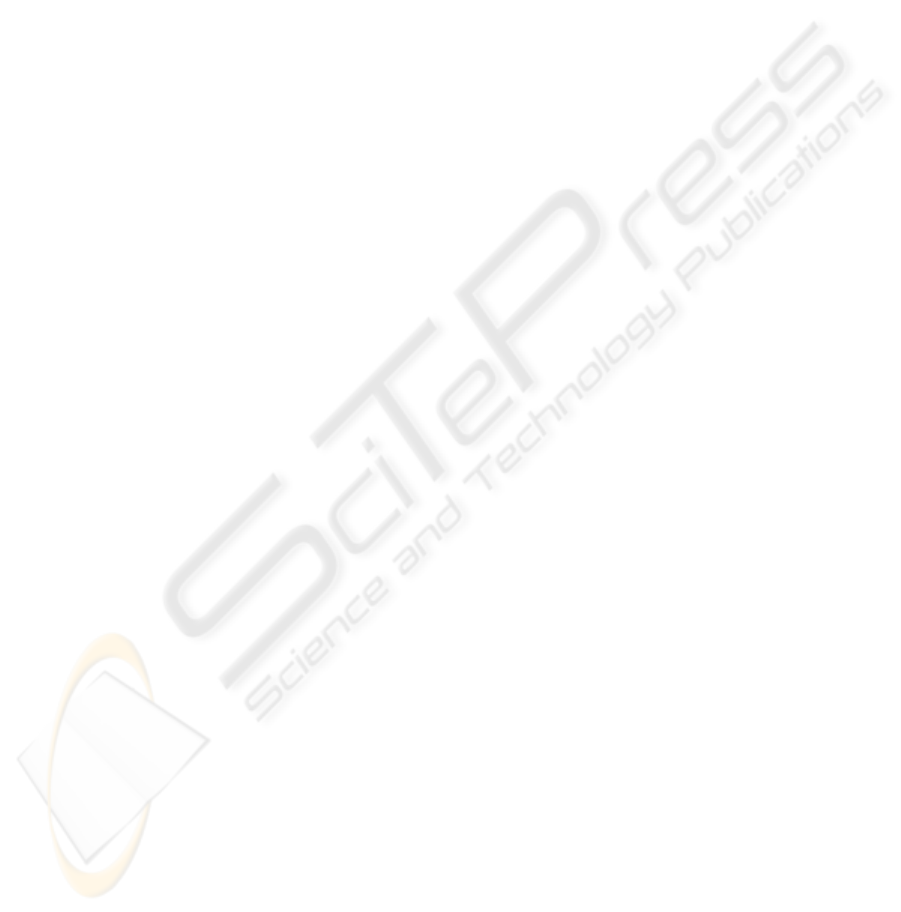
A further application in the field of systems
radiation biology is to investigate the influence of
low-dose irradiation on cell-cell interactions and
possible bystander effects. The medical use of
ionizing radiation contributes the largest fraction to
the population’s anthropogenic radiation exposure.
Thus, biopsies of suspicious diagnostic findings,
which were irradiated with standard low-dose, will
be extracted for histological examination and
isolated cell clusters will be analysed on the LOC.
By spotting a specific protein array on one part of
the LOC and moving cells and media via SAW onto
this particular array, it should be possible to detect
cancer cascades and involved proteins. This method
can be further used to determine the relation and
interaction between cancer associated proteins i.e.
p53, TGF-β and caspase.
5 OUTLOOK
This system, the acoustically driven, freely
programmable multifunctional biochemical lab-on-
a-chip system, will be
applied on different
diagnostic approaches at the single cell or single
chromosome level e.g. cytogenetics, tumour genetics
and genetic forensics.
Competitive LOCs, combining these techniques,
are worldwide not on the market. An essential
advantage of the LOC system is the modular set-up,
which allows reacting to different diagnostic
questions in a preventive medical check-up. This
implements the rapid adaptation to patient-specific
point-of-care diagnostics as well as the operator-
specific development of new molecular markers for
imaging techniques. Fields of applications for the
newly developed LOC system range from the
analysis of cell compartments and single cells in
tumour diagnosis to chromosomal imbalances in the
human genome.
ACKNOWLEDGEMENTS
The authors would like to thank Klaus Macknapp,
Deutsches Museum Munich, for providing the
electron microscopy images.
Financial support of the Bavarian Research
Foundation, Deutsche Forschungsgemeinschaft
(DFG) SFB 486 grant and German Excellence
Initiative via the ‘Nanosystems Initiative Munich
(NIM)’ is gratefully acknowledged.
REFERENCES
Buck M.J. and Lieb J.D., 2004. ChiP-chip: considerations
for the design, analysis, and application of genome-
wide chromatin immunoprecipitation experiments.
Genomics 83: 349-360
Edelmann, L., Hashmi, G., Song, Y., Han, Y., Kornreich,
R., Desnick, R.J., 2004. Cystic fibrosis screening:
validation of a novel method using Bead Chip
technology. Genet Med. 6(5): 431-438
Guttenberg, Z., Müller, H., Habermüller, H., Geisbauer,
A., Pipper, J., Felbel, J., Kielpinski, M., Scriba, J.,
Wixforth, A., 2005. Planar chip device for PCR and
hybridization with surface acoustic wave pump. Lab
on a chip 5: 308-317
Harrison, D.J., Fluri, K., Seiler, K., Fan, Z., Effenhauser,
C.S., Manz, A., 1993. Micromaching a miniaturized
capillary electrophoresis-based chemical analysis
system on a chip. Science 261: 895-897
Ibrahim, M.S., et al., 1998. Real-time microchip PCR for
detecting single-base differences in viral and human
DNA. Anal Chem. 70: 2013
Kwakye, S., Baeumner, A., 2003. A microfluidic
biosensor based on nucleic acid sequence recognition.
Anal. Bioanal. Chem. 376(7): 1062-1068
Lagally, E.T., Emrich, C.A., Mathies, R.A., 2001. Fully
integrated PCR-capillary electrophoresis microsystem
for DNA analysis. Lab on a chip 1: 102-107
Ng, J.H., Ilag, L.L., 2003. Biochips beyond DNA:
technologies and applications. Biotechnol. Annu. Rev.
9: 1-149
Rau, R., 1997. The National Institute of Justice Forensic
DNA Laboratory Improvement Program. Profiles
DNA 1: 5
Schneegass, I., Brautigam, R., Kohler, J.M., 2001.
Miniaturized flow-through PCR with different
template types in a silicon chip thermocycler. Lab
Chip 1(1): 42-49
Thalhammer, S., Lahr, G., Clement-Sengewald, A., Heckl,
W.M., Burgemeister, R., Schütze, K., 2003. Laser
microtools in cell biology and molecular medicine.
Laser Physics 13(5): 681-692
Thalhammer, S., Langer, S., Speicher, M.R., Heckl, W.M.,
Geigl, J.B., 2004. Generation of chromosome painting
probes from single chromosomes by laser
microdissection and linker-adaptor PCR. Chromosome
Research 12: 337-343
Thalhammer, S., von Guttenberg, Z., Koehler, U., Zink,
A., Heckl, W.M., Franke, T., Paretzke, H.G.,
Wixforth, A., 2007. Programmierbares,
zytogenetisches Submikroliter-Chiplabor für
molekular-diagnostische Anwendungen.
GenomXPress ISBN: 1617-562X, 1.07: 29-31
Thorsen, T., Maerkl, S.J., Quake, S.R., 2002. Microfluidic
large-scale integration. Science 298: 580-584
Wixforth, A., Strobl., C., Gauer, C., Toegl, A., Scriba, J.,
Guttenberg, Z., 2004. Acoustic manipulation of small
droplets. Anal. Bioanal. Chem. 379: 982-991
PROGRAMMABLE CYTOGENETIC SUBMICROLITRE LAB-ON-A-CHIP FOR MOLECULAR DIAGNOSTIC
APPLICATIONS
261