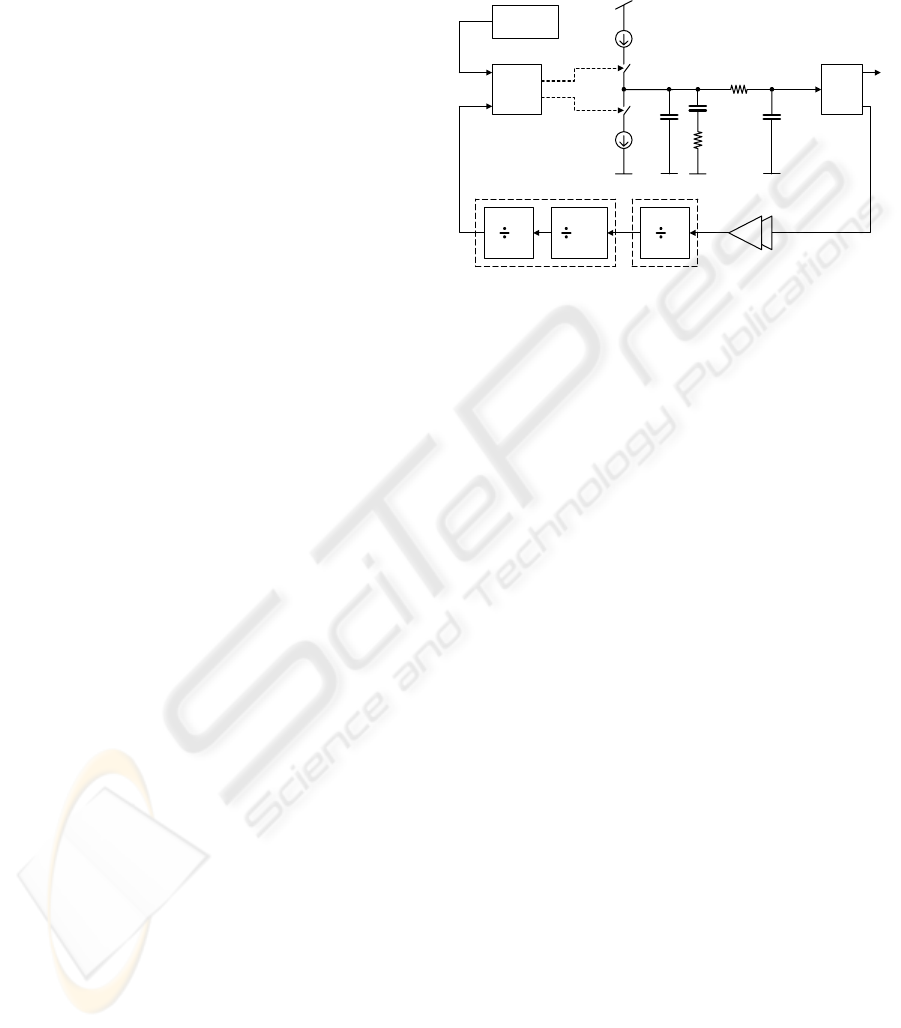
2.3 Frequency Synthesizer
As depicted in Figure 4, the PLL has a reference
generator circuit with a crystal based oscillator at
20 MHz, followed by a phase-frequency difference
circuit (PFD) without dead zone, a current steering
charge pump (CP) and a third order passive filter.
The passive section output is connected to the VCO,
which generates the desired frequencies of 2.4 GHz
or 2.48 GHz. These frequencies must be divided by
120 or by 124 and connected to the PFD again,
closing the loop. The frequency division ratio is
selected by the one bit control signal 120/124. This
selects the value of the frequency to be connected to
the mixer, in order to make the up or the
down-conversion operation.
The division by 120/124 in the feedback path is done
with a cascade constituted by one half divider
implemented with a true single phase clock (TSPC)
logic, one divider by 30/31 (digitally selected by the
signal 120/124), followed by a toggle flip-flop to
ensure a duty-cycle of 50% at the PFD input. The
TSPC logic was used to overcome the impossibility
to implement the first toggle flip-flop with static
logic in this technology. It is required a rail-to-rail
input to work properly. Moreover, at these
frequencies, the power consumptions are lowest
compared with the SCL logic (Pellerano et al, 2004).
The ratios of 30 and 31 were achieved with the use
of frequency dividers by 2/3 with modulus control.
3 WIRELESS ELECTRONIC
SHIRT
Like any other every-day garment piece, the wireless
electronic shirt (WES) will be lightweight, machine
washable, comfortable, easy-to-use shirt with
embedded sensors. To measure respiratory and
cardiac functions, sensors are plugged into the shirt
around patient’s chest and abdomen. The WES also
uses small sizes and compact modules, made with
microsystems, containing the 2.4 GHz RF CMOS
transceiver, the electronic of control and processing,
and the interfaces to make the connections to the
sensors. These modules have also an associated
antenna and are supplied by a coin-sized battery.
The size-reduction achieved with these modules,
make them suitable to be easily plugged in the WES,
according the interest of the medical doctors.
Conventional applications of electronic shirts uses
wires to connect the sensors to a central unit, and
then to make the RF communication to the external
base-station (Marculescu et al., 2003). An
interesting application and an easy way to
implement wireless buses, is using modules able to
communicate between them and between the
base-station.
Reference
generator
PFD
VCO
F
ref
F
div
Up
Down
I
cp
I
cp
C1 C2
R2
C3
R3 V
control
Buffers
F
out
2
Static logic
30/31
2
TSPC
Figure 4: The structure of the PLL.
A single channel measures heart rate and an
inductive elastic band will be used for monitoring
the respiratory function.
It is used a bending sensor to monitor the shoulders
positions, that suffers changes in the electrical
resistance when it is bent. As this sensor is bent the
resistance gradually increases. When the sensor is
bent at 90 degrees, its resistance will range between
from the 30 kΩ up to the 40 kΩ. It was also used an
elastic band to measure the changes in thoracic
circumference due to respiration. The transducer
contains an variable inductance, used indirectly to
measure the changes in thoracic circumference. The
device should be placed around the body at the level
of maximum respiratory expansion. This level will
change between erect and supine positions. At
maximum inspiration the belt should be stretched
almost to maximum extension, making its
inductance minimum. As shown in the Figure 5, the
PLL reference oscillator is reused, to generate the
20 MHz sinusoidal signal, required for this circuit.
The variations in the inductance, changes the
attenuation for the 20 MHz signal, in the first RL
low-pass of first order filter. Thus, the attenuation
increases with the decreasing of the thorax
perimeter. This filtered signal is further amplified,
before a second low-pass filtering, to eliminate noise
and spurs generated in the 20 MHz oscillator. Then,
a peak detector gets the amplitude of the 20 MHz
processed signal. This amplitude is a low-frequency
signal, and it is converted to the digital domain,
using a ΣΔ analog-to-digital converter (ADC) of first
order, with an output with resolution of eight bits.
This allows the recording of respiratory changes
with maximum sensitivity and linearity.
BIODEVICES 2008 - International Conference on Biomedical Electronics and Devices
164