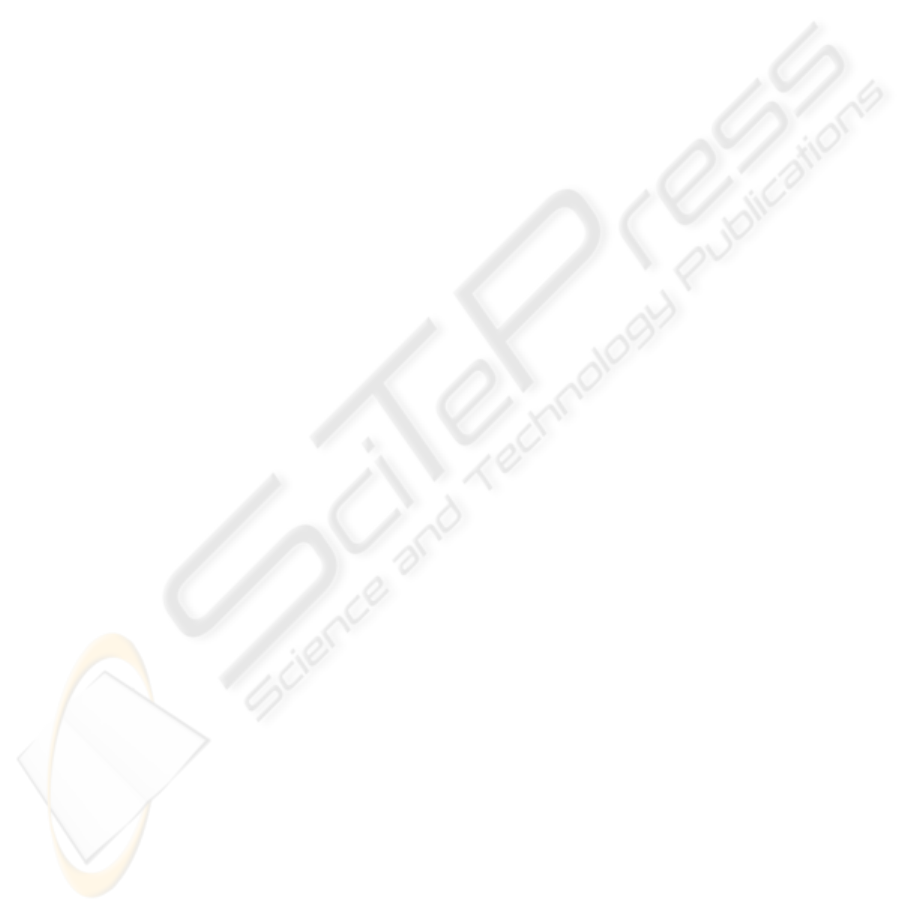
triceps decreased while the contribution of the
biceps increased, but even so the biceps EMG signal
remained smaller than the triceps EMG signal. This
situation changed at half of the movement, where the
elbow was in a full flexed position, requesting more
of the biceps.
On the other side, for the movements performed
at the vertical plane, EMG signal from the biceps
showed greater intensity at the beginning and at the
end of the movement (intervals from 0º to 10º and
from 10º to 0º). The gravity influence is greater over
the biceps at this plane. To surpass inertia the
requested muscle was not triceps, as verified at the
horizontal plane, but it was the biceps. Quantitative
analysis showed that the intensity of EMG signal of
the triceps was quite constant at this plane.
Aiming to quantify the variability of data sets
during the movement and among each repetition
made by the same volunteer, coefficients of
variation were calculated. According to Araújo
(2000), the coefficient of variation from the EMG
signal is very high, being around 21.61% the
average of variation of that parameter. It means that
values around 20% do not indicate, necessarily,
different muscular activities. This high variation can
be caused by factors as position and quality of the
electrodes, change of temperature and changes in the
metabolism.
The coefficients of variation values obtained
from the tests performed at the horizontal plane were
around 25%. Therefore, it can be considered that
there was repeatability of the movements performed.
Moreover the repeatability inter the several
movements executions made by the same volunteer,
the existence of a standard pattern of EMG
intensities for both muscles, certify that there is a
repeatability intra volunteers and also the existence
of a recruitment pattern.
Analyzing the coefficients of variation obtained
from the movements performed at vertical plane, the
values related with the triceps were around 25%,
indicating the same pattern analyzed previously. By
the other side, although the coefficients related with
the biceps, for each repetition was small, indicating
little variation during the test, the values between
repetitions were around 35%, indicating that there
was no repeatability among recruitment for each
movement performed by the same volunteer. There
were cases with coefficients above 50% that
probably were related with some isolated stronger
muscular contraction made during the test. At
vertical plane it was not possible to identify a
recruitment pattern due to the variability of the data.
Besides that, it was observed small
displacements of electrode positions during
movements, and it must be
also considered
interference due the signals of adjacent muscles.
These comments are in accordance with verified by
DeLuca (1997), in other experiments.
5 CONCLUSIONS
During the tests, the muscle triceps showed a quite
constant behavior, with repeatability of movements
at both planes. The muscle biceps demonstrated this
behavior only for movements performed at the
horizontal plane, in which it had a little recruitment.
For movements performed at the vertical plane, the
biceps was more requested, resulting in a greater
intensity EMG signal, but without repeatability.
There are several factors that can disturb EMG
signal, but despite of the interference, it is possible
to characterize movements by means of EMG,
showing the intensity of muscle recruitment.
The results showed the existence of a recruitment
pattern for biceps and triceps among different
subjects but only for movements performed at the
horizontal plane.
ACKNOWLEDGEMENTS
The authors would like to thank FAPESP and FEI
support.
REFERENCES
Araujo, R. C., Duarte, M., & Amadio, A. C. (2000). On
the inter- and intra-variability of the
electromyographic signal in isometric contractions.
Eletromyogr. Clin. Neurophysiol., 40: 225-229.
Capucho, A. C. (2005). Desenvolvimento de um Protótipo
de um Aquisitor Portátil de Sinal Mioelétrico.
Dissertação de Mestrado em Bioengenharia,
Universidade se São Paulo, São Carlos.
DeLuca, C. J. (1979). Physiology and Mathematics of
Myoelectric Signals. IEEE Trans. Biom. Eng., 26:
313-325.
DeLuca, C. J. (1997). The use of surface
electromyography in biomechanics. J. Appl. Biomech.,
13(2): 135-163.
DeLuca, C. J. (2002). Surface Electromyography:
Detection and Recording. DelSys Inc.
Guyton, A. C., & Hall, J. E. (2002). Fundamentos de
Guyton, Tratado de Fisiologia Médica. Guanabara,
Rio de Janeiro.
BIODEVICES 2008 - International Conference on Biomedical Electronics and Devices
150