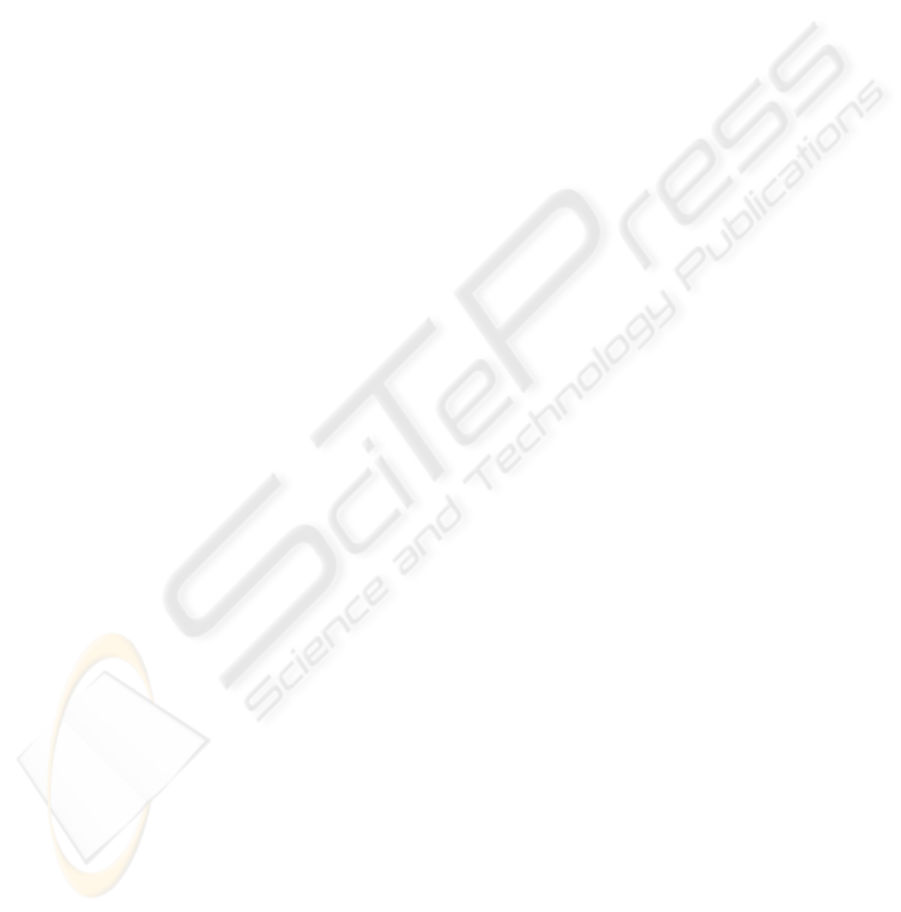
vein valve in an in vitro blood set-up is a novel
process. The most common practice to test the
thrombotic potential is in an animal model, where
eight out of ten studies reviewed failed due to in vivo
thrombosis. The two most successful vein valve
studies use acellular tissues: the SG-BVV was
constructed from porcine small intestine submucosa
(SIS), and the PVVB used gluteraldehyde-preserved
bovine jugular valves (Moll, 2003), (Gale et al,
2004). The GT vein valve provides several
advantages over SIS and gluteraldehyde-preserved
bovine jugular valves. Zoonosis from animal tissue
prosthetics is possible and the use of animal derived
prosthetics may be culturally or religiously
controversial, therefore a synthetic material would
alleviate these concerns. The PVVBs are fixed with
gluteraldehyde, which is a toxic substance that will
prevent cells from integrating into the material in
vivo. This gluteraldehyde preservation process will
cause a limited cyclic life due to the cross-linking of
the collagen fibers, and ongoing biocompatibility
issues due to the gluteraldehyde toxicity. The SIS
tissue appears to be an appropriate material for vein
valve prosthetics with regards to its
biocompatibility. However, despite revisions to the
SIS vein valve, the SG-BVV continues to experience
in vivo tilting. Tilting is not an issue with the GT
vein valve because of the long axial dimension. In
addition, GT vein valve can be mass-produced and
the design is easily modified. This is unlike acellular
tissues which require extensive tissue preparation
and processing times and modification of the tissue
valve design could create concerns regarding
suturing locations and tissue-to-stent attachment
sites.
Another feature of the new GT Valves is that
they may be processed to include embedded drugs,
which could promote cell growth and/or reduce
thrombus formation. It has superior biocompatibility
and structural integrity, may be mass-produced, and
has the potential to utilize new drug delivery
technologies.
Providing relief to chronic venous insufficiency
is a worthwhile pursuit as patients experience
swelling, edema, pain, itching, varicose veins, skin
discoloration, ulceration and limb loss. Current
clinical therapies are only modestly effective; and
therefore, a prosthetic vein valve may provide a cure
for this debilitating disease. With successful animal
and human trials this valve could provide a useful
therapy the 7 million people suffering from chronic
venous insufficiency. The GT valve exhibits
excellent flow, full competency, fatigue-resistance,
low-thrombogenicity, material flexibility, and in situ
placement consistency.
REFERENCES
de Borst, G.J., et al., A percutaneous approach to deep
venous valve insufficiency with a new self-expanding
venous frame valve. Journal Of Endovascular Therapy,
2003. 10(2): p. 341-349.
Gale, S.S., et al., Percutaneous venous valve
bioprosthesis: initial observations. Vascular And
Endovascular Surgery, 2004. 38(3): p. 221-224.
Gomez-Jorge, J., A.C. Venbrux, and C. Magee,
Percutaneous deployment of a valved bovine jugular
vein in the swine venous system: a potential treatment
for venous insufficiency. Journal Of Vascular And
Interventional Radiology: JVIR, 2000. 11(7): p. 931-
936.
Hill, R., et al., Development of a prosthetic venous valve.
Journal of biomedical materials research., 1985. 19(7):
p. 827.
Moll, F. Venous Valves for Chronic Venous Insufficiency.
in Vascular and Endovascular Controversies. 2003.
London, UK.
Pavcnik, D., et al., Significance of spatial orientation of
percutaneously placed bioprosthetic venous valves in
an ovine model. Journal Of Vascular And
Interventional Radiology, 2005. 16(11): p. 1511-1516.
Rosenbloom, M.S., et al., Early experimental experience
with a surgically created, totally autogenous venous
valve: a preliminary report. Journal of vascular
surgery, 1988. 7(5): p. 642.
Sathe, R.D. and D.N. Ku, Design and Development of a
Novel Implantable Prosthetic Vein Valve. J Med
Devices, 2007. 1: p. 105-112.
Taheri, S.A. and R.O. Schultz, Experimental prosthetic
vein valve. Long-term results. Angiology., 1995.
46(4): p. 299.
Uflacker, R. Percutaneously introduced artificial venous
valve: Experimental use in pigs. in The 1993 Annual
Meeting of the Western Angiographic & Interventional
Society. 1993. Portland, OR.
PRECLINICAL TESTING OF A NEW VENOUS VALVE
35