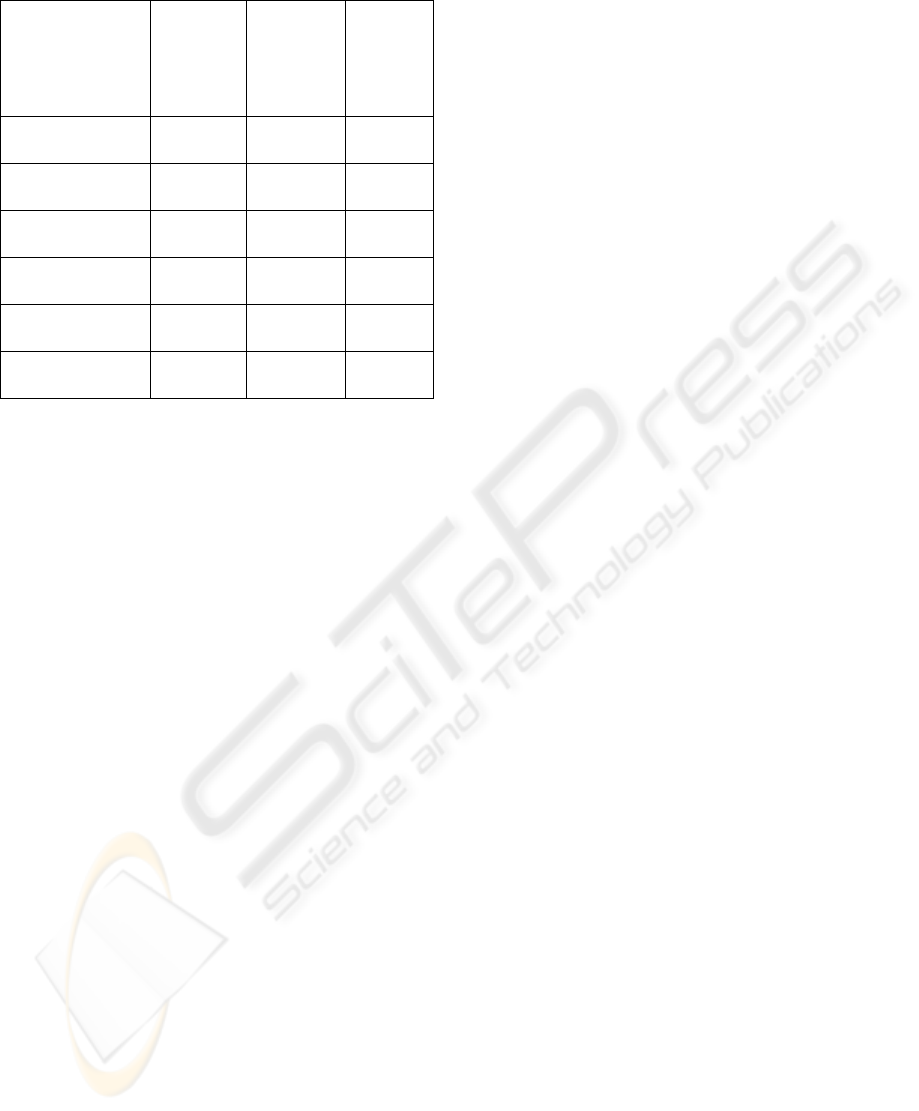
Table 2: Comparison of performance.
Configuration Duration
(hardware)
[no. of
clock
cycles]
Running
speed*
(hardware)
[seconds]
Running
speed
(software)
[seconds]
1 outer turn 40,960 0.0004
7
4.2
2 outer turns 163,84
0
0.0019
4
18
4 turns
(2 out. 2 in.)
655,36
0
0.0076
4
72
Slinky_1 coil 4,096,
000
0.0470
5
420
Slinky_2 coil 16,384
,000
0.1941
1
1,680
Slinky_3 coil 36,864
,000
0.4294
1
3,600
* at 85.714 MHz, clock period 11.66 ns
As a global comparison, the hardware solution
runs approximately four orders of magnitude faster
then the software one. The frequency is related to
the physical board we had available, but for a more
recent FPGA chip (i.e. Virtex4LX160, for which we
did some simulations, or Virtex5), the device’s
capacity as well as the working frequency will
increase, thus leading to an improved performance.
5 CONCLUSIONS AND FUTURE
WORK
An adequate geometry of the stimulation coil can
lead to a better focality of the stimulus (the ability of
a coil to stimulate a small area of the tissue) and it
can also improve the efficiency of the energy
transfer from the coil to the target tissue. The form
and size of the turns, their position inside the coil,
the insulation gap between turns are all important
parameters that should be considered when
designing a magnetic coil. Therefore, in order to
establish the most suitable coil geometry for a
specific medical application, a large number of
structures have to be tested, making of coil design a
trial-and-error process, even if the risk involved is
only computation time.
In (Creţ et. al., 2007), we analyzed the influence
that space distribution of the magnetic coils’ turns
has on the efficiency of energy transfer from the
stimulator to the target tissue. The analysis was
performed for a Slinky_3 coil configuration, with
applications on transcranial magnetic stimulation
(TMS). It turned out that the electrical energy
dissipated in the circuit of the stimulator – required
in order to achieve the activation threshold – is 25%
lower for the most efficient configuration than for
the less efficient one, and the coil heating per pulse
is also 35% smaller!
This estimation was based on the inductivity
calculus described in this paper, and the large
number of analyzed structures required a less time-
consuming computation technique, the hardware
implementation described above.
Since every medical application requires its own
optimal structure of the magnetic coil, the results
emphasized in this paper can play an important role
for future work on coil design.
Because of the large amount of operations
involved (several tens of millions just for one coil) it
is very hard to debug such a hardware system at
least at an acceptable level, but the obtained results
show an excellent concordance with those obtained
in software. Our implementation has the advantage
of greatly speeding up the computation time and
hence shortening the design process. On larger
FPGA devices the process can achieve a greater
speed by accommodating more computational
structures in parallel. These structures would
evaluate multiple pairs of segments in parallel and
accumulate them to the final value.
REFERENCES
Collange, S., Detrey, J., & De Dinechin, F. (2006).
Floating Point or LNS: Choosing the Right Arithmetic
on an Application Basis. In Proceedings of the 9
th
EUROMICRO Conference on Digital System Design,
Dubrovnik, Croatia, 197-203.
Creţ, L., Pleşa, M, Micu, D.D., & Ciupa, R. (2007).
Magnetic Coils Design for Focal Stimulation of the
Nervous System. In Proceedings of EUROCON 2007,
IEEE International Conference on Computer as a
Tool, Warsaw, Poland, 1998-2003.
Detrey, J, & De Dinechin, F. (2005). A Parameterizable
Floating-Point Logarithm Operator for FPGAs. In
Proceedings of the 39
th
Asilomar Conference on
Signals, Systems & Computers, 1186 – 1190.
Detrey, J, & De Dinechin, F. (2007). FPLibrary.
https://lipforge.ens-lyon.fr/projects/fplibrary/.
Griškova1, I., & Höppner, J. (2006). Transcranial
magnetic stimulation: the method and application.
Medicina (Kaunas), 42(10), 792-804.
Guell, D., El-Ghazawi, T., Gaj, K., & Kindratenko, V.
(2007). High-Performance Reconfigurable Computing,
IEEE Computer, 40 (3), 23-27.
Mozeg, D., & Flak, E. (1999). An Introduction to
Transcranial Magnetic Stimulation and Its Use in the
Investigation and Treatment of Depression. University
of Toronto Medical Journal, 76 (3), 158-162.
FPGA-BASED COMPUTATION OF THE INDUCTANCE OF COILS USED FOR THE MAGNETIC STIMULATION
OF THE NERVOUS SYSTEM
155