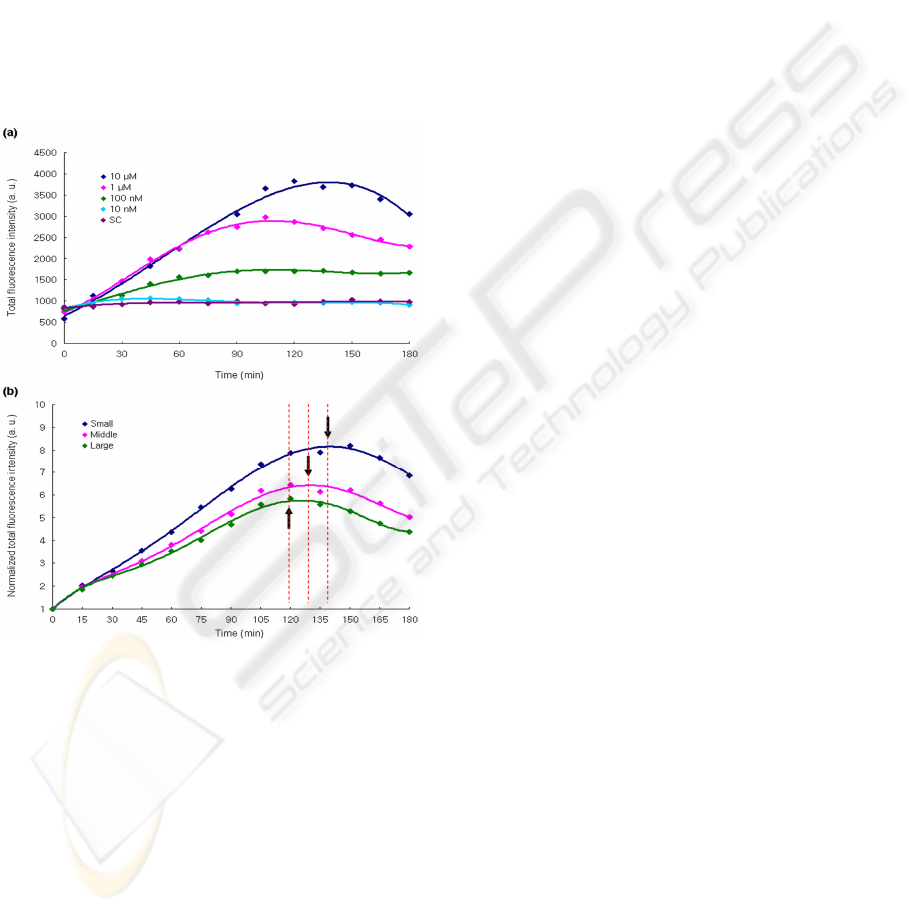
with EGFP and Tdimer2 reporters driven by the α-
factor-responsive P
Fus1
promoter or by the α-factor-
independent P
Gpd1
promoter (i.e., SH129), the total
fluorescence intensity, noise and noise strength upon
stimulation of 10 µM α-factor are characterized as
shown in Figure 6.
Figure 7 shows the stochastic gene expression in
SH133 strain which contain C-terminal residues
Cln2 (yeast G1 cyclin) PEST motifs. The Cln2
(PEST) destabilized EGFP so that it allows dynamic
monitoring of transcription over time. Figure 7a
shows dose-dependent gene expression of P
Fus1
-
EGFP upon stimulation of α-factor. Interestingly, the
mating MAPK signaling has different kinetic gene
expressions as increasing cellular area (Fig. 7b).
Figure 7: Analysis of stochastic gene expression in
SH133.
Similarly, the SH135 strain whose character is
protein localization exhibits the stochastic gene
expression (not shown).
3 CONCLUSIONS
We have presented an optimal experimental setup
for analyzing gene expression dynamics which
would be capable of both monitoring the behavior of
a large population of cells and of tracking individual
cells. It was composed of yeast strain construction,
single-cell docking, automated image acquisition,
extraction of quantitative information, analyzing and
modelling of the stochastic gene expression. Using
this cell chip platform, we could successfully have
an insight into the stochastic nature of gene
expression, so we hope that many other investigators
also will have such insight more easily aided this
high-throughput and high-content single-cell
analysis method.
REFERENCES
Ashkin, A. (1997). Optical trapping and manipulation of
neutral particles using lasers. Proceedings of the
National Academy of Sciences of the United States of
America, 94(10), 4853-4860.
Biran, I., & Walt, D. R. (2002). Optical Imaging fiber-
based single live cell arrays: A high-density cell assay
platform. Analytical Chemistry, 74(13), 3046-3054.
Elowitz, M. B., Levine, A. J., Siggia, E. D., & Swain, P. S.
(2002). Stochastic gene expression in a single cell.
Science, 297(5584), 1183-1186.
Figeys, D., & Pinto, D. (2000). Lab-on-a-chip: A
revolution in biological and medical sciences.
Analytical Chemistry, 72(9), 330a-335a.
Hertzberg, R. P., & Pope, A. J. (2000). High-throughput
screening: new technology for the 21st century.
Current Opinion in Chemical Biology, 4(4), 445-451.
Khademhosseini, A., Suh, K. Y., Jon, S., Eng, G., Yeh, J.,
Chen, G. J., et al. (2004). A soft lithographic approach
to fabricate patterned microfluidic channels.
Analytical Chemistry, 76(13), 3675-3681.
Park, M. C., Hur, J. Y., Kwon, K. W., Park, S. H., & Suh,
K. Y. (2006). Pumpless, selective docking of yeast
cells inside a microfluidic channel induced by
receding meniscus. Lab on a Chip, 6(8), 988-994.
Raser, J. M., & O'Shea, E. K. (2004). Control of
stochasticity in eukaryotic gene expression. Science,
304(5678), 1811-1814.
Reyes, D. R., Iossifidis, D., Auroux, P. A., & Manz, A.
(2002). Micro total analysis systems. 1. Introduction,
theory, and technology. Analytical Chemistry, 74(12),
2623-2636.
Suh, K. Y., Kim, Y. S., & Lee, H. H. (2001). Capillary
force lithography. Advanced Materials, 13(18), 1386-
1389.
Voldman, J., Gray, M. L., Toner, M., & Schmidt, M. A.
(2002). A microfabrication-based dynamic array
cytometer. Analytical Chemistry, 74(16), 3984-3990.
Wheeler, A. R., Throndset, W. R., Whelan, R. J., Leach,
A. M., Zare, R. N., Liao, Y. H., et al. (2003).
Microfluidic device for single-cell analysis. Analytical
Chemistry, 75(14), 3581-3586.
YEAST ON A CHIP - Single-cell Analyses of MAPK Signaling Pathways in Saccharomyces Cerevisiae using Cell Chips
271