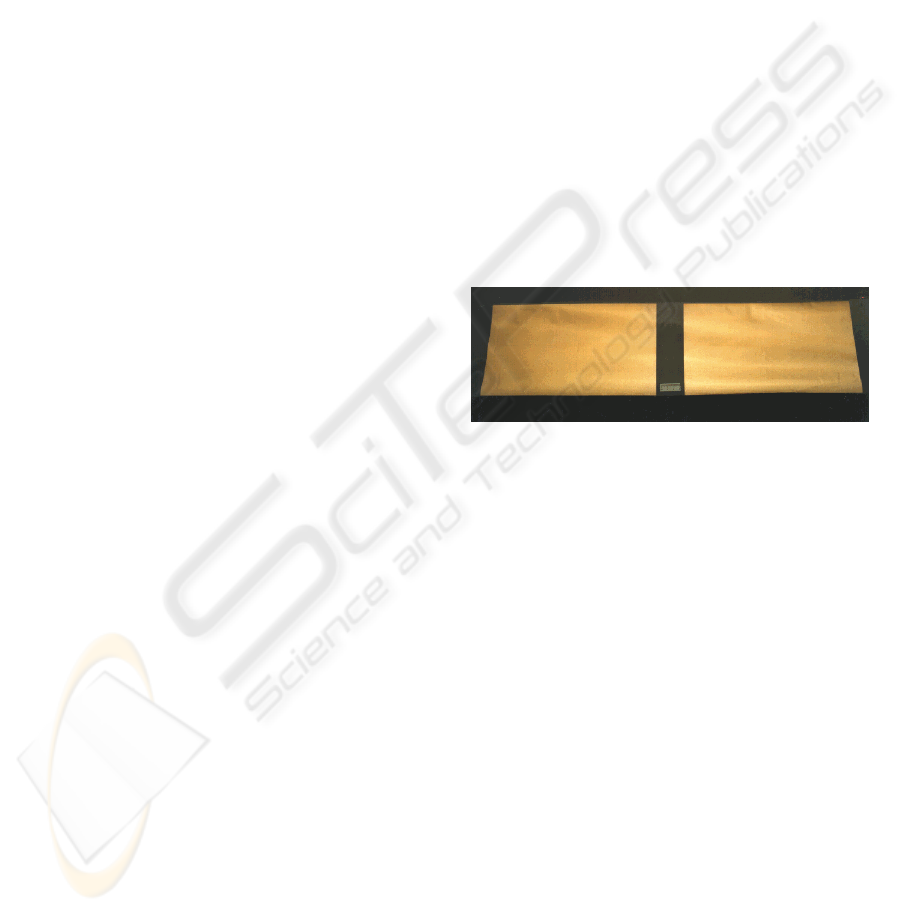
distinguish bruxism episodes from mioclonus or
rapid contractions (< 0.5 s) of the jaw muscles.
However, it is important to point out that
everybody subconsciously clenches their teeth at
some time of the day and this could be considered as
bruxism activity. The term bruxism is only used
though when the duration and intensity of this
activity has a bearing on dental wear and the
appearance of TMJ problems.
One of the main problems associated with the
traditional diagnosis of bruxism is that it is
frequently made when the teeth are already highly
worn and the prognosis of the illness is more severe.
Bruxism activity can also be recorded by an EEG
(electroencephalogram) as well as by EMG
(electromiography) and S-EMG (surface
electromiography). In many cases video-cameras are
used in the study to distinguish the bruxism episodes
of the mioclonus or rapid contractions (< 0.5 s) of
the jaw muscles.
However, in order to be able to make an earlier,
more rapid, more effective and economical diagnosis
of bruxism, the research team that have written this
paper propose using instrumented splints for
detecting and recording the intensity and duration
of interdental pressure episodes. Explained below
are the design, manufacture and trials of an
instrumented splint for the diagnosis of bruxism
activity. It has been developed by researchers at
Universidad Politécnica de Madrid in collaboration
with Ibex Estética Dental S.L..
2 DESIGN OF THE DEVICE FOR
DIAGNOSING BRUXISM
USING ELECTROACTIVE
POLYMERS AS SENSORS
Traditionally discharge splints or protection devices
are used to treat bruxism and prevent the associated
dental wear. As a diagnostic device we propose
introducing pressure sensors into a splint so that
patients’ bite episodes can be recorded and the
extent of their pathology be assessed. Piezoelectric
polymers are used as pressure sensors for the
reasons set out below.
Piezoelectric Electroactive Polymers as
Pressure Transducers: PVDF (Polyvinylidene
Fluoride)
Piezoelectricity was discovered in 1880 by
Pierre and Paul-Jaques Curie, who observed that
when certain crystals were compressed, like quartz
or tourmaline, depending on certain directions they
produced a voltage between zones on their surface.
When force was applied the relative positions of the
crystal molecules changed producing an internal
displacement of charges which was the cause of this
voltage.
These crystals also underwent the inverse effect
since they became deformed when a voltage
difference was applied. This property is found in
materials lacking a centre of symmetry and the
phenomenon is called ferroelectricity when a non-
conductor crystal or dielectric material exhibits
spontaneous electric polarisation.
Polyvinylidene fluoride or PVDF -(CH
2
-CF
2
)-
n
and its co-polymers such as poly(vinilydenefluoride-
trifluoroethylene) or P(VDF-TrFE), are the polymers
of this kind with the largest number of industrial
applications. They posses partial crystalinity with an
inactive amorphous phase and an elastic modulus
close to between 1 and 10 GPa. Their use as
actuators is limited by the need to apply high electric
fields (around 20 V/μm for a 3% deformation), but
their use as pressure sensors is taking the place of
traditionally used piezoelectric ceramic materials.
Figure 1: Metallized PVDF sheets. Piezotech S.A..
The use of this type of sensor was considered
because of its reduced thickness, which does not
cause any alteration to the patient’s bite and because
of its greater resistance and sensitivity compared to
ceramic piezoelectrics. To make the sensors, we
took PVDF 40 μm thick sheets from Piezotech S.A.
with Au-Pt coated electrodes. These sheets were cut,
joined to the connecting wires and suitably
encapsulated to protect them and be inserted into the
splint (see manufacturing process). The sensors
obtained are shown below, together with the
behaviour model allowing them to be simulated and
the first results obtained in the trials carried out.
INSTRUMENTED SPLINT FOR THE DIAGNOSIS OF BRUXISM
217