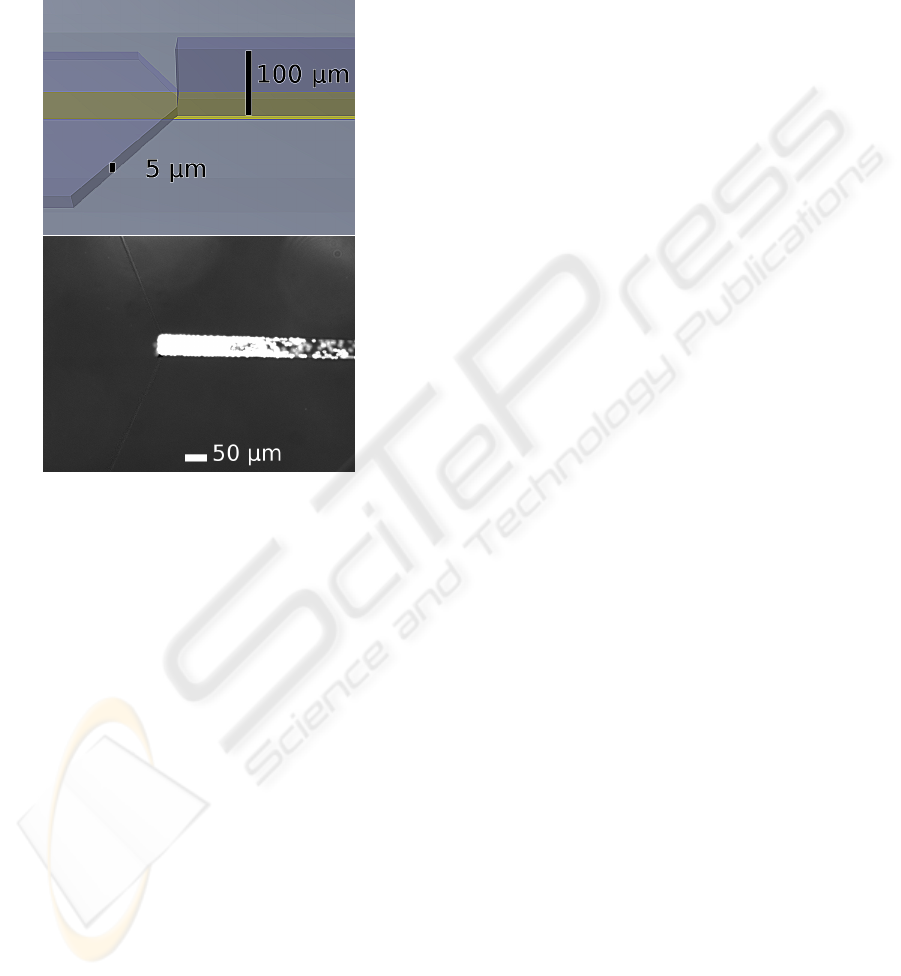
5 µm beads and 4 µm central height. To prevent this,
we injected a limited amount of 10 µm beads prior
to the 5 µm beads, that were prevented from entering
the central part because of the bigger beads. Exper-
iments with PDMS devices and beads modified with
PNIPAM are currently underway.
Figure 7: Top: 3D close-up on the channel zone where
beads are blocked. Bottom: Top-view of fluorescent 10 µm
and 5 µm beads blocked at the entry of the central part.
5 CONCLUSIONS
Thermoresponsive properties of PNIPAM upon tem-
perature are well known and have been demonstrated
as switchable surfaces for protein adsorption. We
demonstrated in this work the possibility to inte-
grate such switchable surfaces into fluidic microsys-
tems dedicated to sample preparation for nanoLC /
ESI-MS. We have developed essential components
and know-how about heating sources, reversible pro-
tein adsorption and release, and injection of beads in
PDMS microchannels. We are now demonstrating the
feasibility of the microsystems for desalting and pre-
concentration of various peptide samples.
REFERENCES
Armani, D., Liu, C., and Aluru, N. (1999). Re-configurable
fluid circuits by PDMS elastomer micromachining. In
Proc. IEEE MEMS ’99.
Gauthier, G. and Grimm, R. (2006). Miniaturization: Chip-
based liquid chromatography and proteomics. Drug
Discov. Today Techn., 3(1):59–66.
Hern
´
andez-Borges, J., Aturki, Z., Rocco, A., and Fanali, S.
(2007). Recent applications in nanoliquid chromatog-
raphy. J. Sep. Sci., 30(11):1589–1610.
Hjert
´
en, S. (1985). High-performance electrophoresis:
Elimination of electroendosmosis and solute adsorp-
tion. J. Chromatogr. A, 347:191–198.
Huber, D., Manginell, R., Samara, M., Kim, B.-I., and
Bunker, B. (2002). Programmed adsorption and re-
lease of proteins in a microfluidic device. Science,
301:352–354.
Ishihama, Y. (2005). Proteomic LC–MS systems using
nanoscale liquid chromatography with tandem mass
spectrometry. J. Chromatogr. A, 1067(1-2):73–83.
Kanazawa, H., Yamamoto, K., Matsushima, Y., Takai,
N., Kikuchi, A., Sakurai, Y., and Okano, T.
(1996). Temperature-responsive chromatography
using poly(n-isopropylacrylamide)-modified silica.
Anal. Chem., 68(1):100–105.
Paumier, G., Sudor, J., Coll
´
e, E., Marty, B., Bancaud, A.,
Camps, T., and Gu
´
e, A.-M. (2007). Electrokinetic
mixers based on stimuli-responding surfaces. In Proc.
11th Int. Conf. on Miniaturized Systems for Chemistry
and Life Sciences (µTAS’2007).
Peterson, D., Rohr, T., Svec, F., and Fr
´
echet, J. (2003).
Dual-function microanalytical device by in situ pho-
tolithographic grafting of porous polymer monolith:
integrating solid-phase extraction and enzymatic di-
gestion for peptide mass mapping. Anal. Chem.,
75(20):5328–5335.
Sudor, J., Paumier, G., Gu
´
e, A.-M., Vinet, F., Est
`
eve, A.,
and Djafari-Rouhani, M. (2006). Spatio-temporal tun-
ing of stimuli-responding surfaces for dynamic con-
trol of electroosmotic flows. In Proc. 10th Int. Conf.
on Miniaturized Systems for Chemistry and Life Sci-
ences (µTAS’2006).
Wilm, M. and Mann, M. (1996). Analytical properties of the
nanoelectrospray ion source. Anal. Chem., 68(1):1–8.
THERMORESPONSIVE POLYMER-BASED MICRODEVICE FOR NANO-LIQUID CHROMATOGRAPHY
181