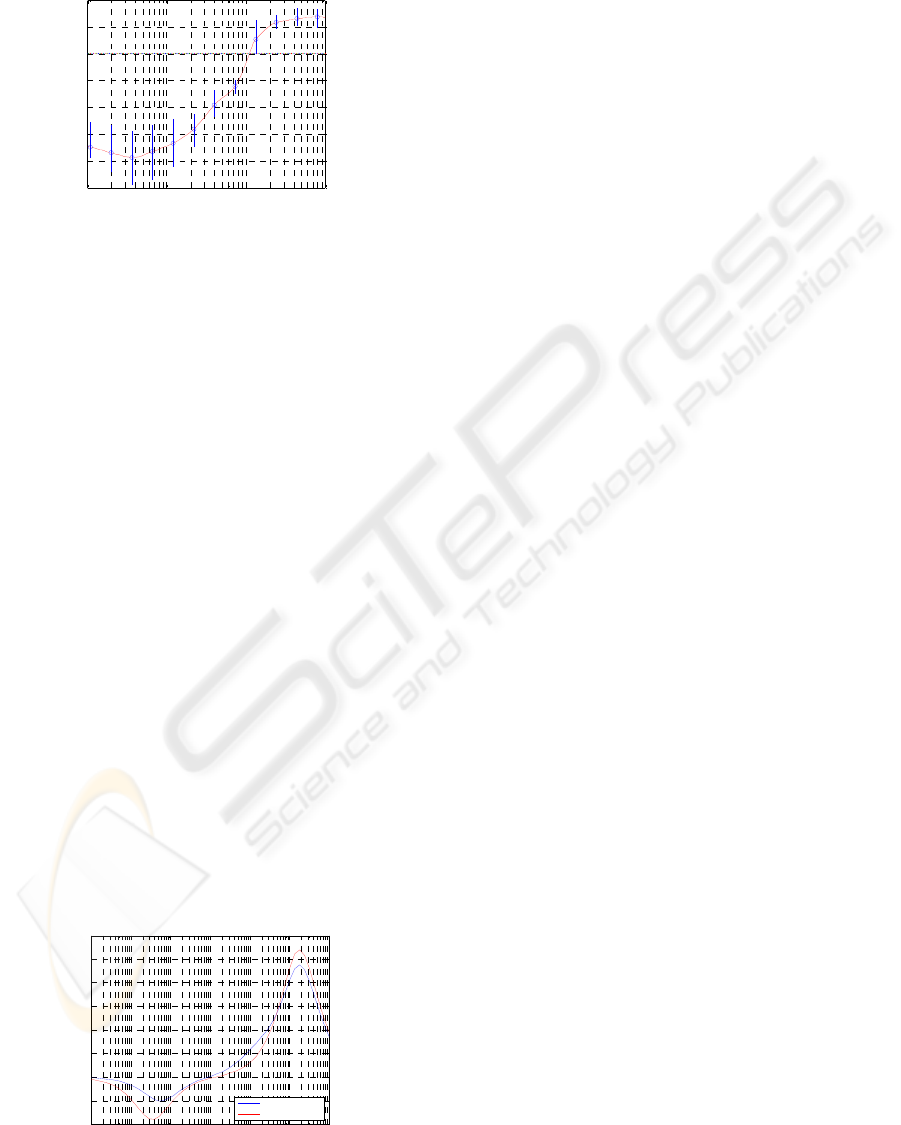
The cells exhibited anti-field rotation at
frequencies below the zero crossing and co-field
rotation above.
10
5
10
6
10
7
10
8
-0.5
-0.4
-0.3
-0.2
-0.1
0
0.1
0.2
f
Hz
ROT rate (rad.s
-
1.V
-
2)
yeast cells protoplasts
Figure 4: Experimental ROT spectra at 50 mS.m
-1
.
4.3 Discussion
For whole yeast cells, the overall spectrum (Figure
3) is consistent with that obtained from simulation
(Figure 2), up to a multiplicative scalar factor, which
could be explained by the fact that the simulated
data did not take into account the factor χ (5).
On both experimental spectra, the negative ROT
peak happens near 500 kHz despite rotation rate
attenuation for yeast protoplasts. The viscosity of
the medium suspension, in the case of protoplasts,
was almost 5% higher than water viscosity because
of glucose (Easteal, 1989), which affects the value
of χ. Nevertheless, more precise investigation is
necessary to understand this rotation slowdown.
As can be seen from Table 1, the dielectric
properties of cell wall are close to medium
properties. This may explains the resemblance
between yeast cells and yeast protoplast simulated
spectra (Figure 2) obtained with our approach,
consisting in switching from a model to another by
suppressing the shell representing the cell wall.
The approach consisting in modelling protoplasts
by the two most inside shells presents its limitations.
Indeed, simulated result does not fit the
experimental data well in the co-field rotation part
of the spectra. For 1.1 mS.m
-1
, simulation points out
more differences between the two spectra (Figure 5).
10
3
10
4
10
5
10
6
10
7
10
8
10
9
-0.2
-0.1
0
0.1
0.2
0.3
0.4
0.5
.
Frequency (Hz)
Imaginary part of Claus ius-Mossotti factor
normal yeast cells
yeas t protoplasts
5 CONCLUSION
As further developments, we need to improve our
experimental setup to cover a wider frequency range
and obtain thereby a complete ROT spectrum,
including the second peak. Future experiments
performed in lower conductivity immersion media
(1.1 mS.m
-1
, for example) may bring more
information about the cell wall influence (Figure 5).
Yeast protoplast and whole cell electric
properties can be extracted from experimental ROT
spectra by parameter identification thanks to a
identification process under Matlab
®
. During this
step, more sophisticated models could be used to
describe cells, as for instance a N-shell ellipsoidal
model. The measurement of cell properties is a step
toward the modelling of electromagnetic field-tissue
interaction using a bottom-up approach.
LabView
®
interface allows to realize several
series of different experiments. Cell motion was
successfully observed over a wide frequency range
for yeast cells. Fabrication of microelectrodes
enabling travelling-wave dielectrophoresis is the last
part of our platform to be developed. Further, these
cell manipulation techniques permit to study the
effects of various treatments on cells such as
response to toxicants for magnetic field exposure
and to detect cell pathologies.
REFERENCES
Kofod, L.V., Andersen, L.N., Kauppinen, M.S., Christgau,
N.S., Dalboge, H., 1998. Enzyme with P-1,6
endoglucanase activity, United States Patent. Patent
Number 5770406.
Falokun, C.D., Markx, G.H., 2006. Electrorotation of
beads of immobilized cells, J. of Electrostatics.
Zhou, X-F., Markx, G.H., Pethig, R., 1996. Effect of
biocide concentration on electrorotation spectra of
yeast cells, Bioch. and Biophys. Acta.
Gascoyne, P. R. C., Vykoukal, J. V., 2004.
Dielectrophoresis-based sample handling in general-
purpose programmable diagnostic instruments,
Proceedings of the IEEE.
Hughes, M.P., 1998. Computer-aided analysis of
conditions for optimizing pratical electrorotation,
Physics in Medecine and Biology.
Hölzel, R., 1997. Electrorotation of Single Yeast Cells at
Frequencies Between 100Hz and 1.6GHz, Biophysical
J.
Easteal, A.J., 1989. Can. Tracer diffusion in aqueous
sucrose and urea solutions, J. Chem..
Fi
ure 5:
Im K
−ω
at 1.1 mS.
-1
.
AUTOMATED CELL CHARACTERIZATION PLATFORM: APPLICATION TO YEAST PROTOPLAST STUDY BY
ELECTROROTATION
193