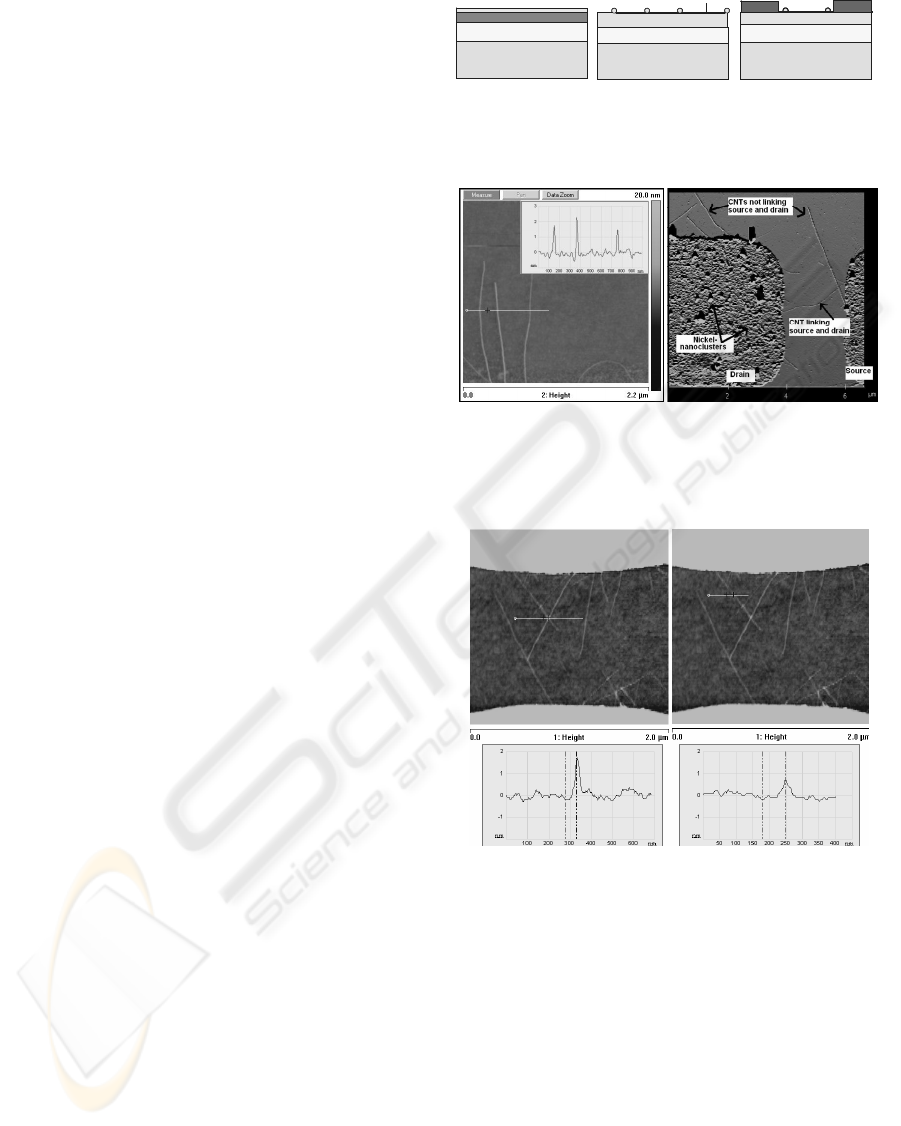
the CNTs (Kong 200; Someya 2003; Staii 2005).
Obviously, commercial large scale integration
remains a major challenge to the realization of CNT-
based nanoelectronics and nanosensor technology as
well.
At our institute we have developed a novel
process to overcome the limitations of manual
fabrication of CNTFETs and hence CNTFESs as
well (Rispal 2006; Rispal 2007; Schwalke 2007).
Our group has succeeded to develop a simple,
reproducible fabrication process to grow individual
SWNTs and SWNT networks as wells in order to
fabricate fully functional CNTFETs and single-
walled CNT-based field-effect controlled sensors.
In this position paper we will first present a brief
summary of our research results on CNTFETs and
subsequently discuss the use of this technology for
possible biomedical CNT-based sensor applications.
2 RESULTS AND DISCUSSION
2.1 CNTFET & CNTFES Fabrication
The process is based on chemical-vapor-deposition
(CVD) growth of CNTs using an aluminum/nickel
‘sacrificial’ catalyst which transforms itself after
CNT growth into a high-k dielectric (i.e. Al
x
O
y
)
covered with dispersed Ni-nanoclusters (Rispal
2007). SWNTs are grown uniformly across the
wafer surface and subsequently contacted with
palladium for S/D contacts and the Si-substrate acts
as a gate electrode as illustrated in Fig. 1. The
process contains neither complicated manipulations
of the SWNTs nor multi-step lithography and is Si-
CMOS compatible. We choose the in-situ growth
method because it appears the most practical
approach for future use in high-volume fabrication
of advanced integrated nano-sensors at low cost.
For the development of this novel process we
have extensively used atomic force microscopy
(AFM) for process control and to optimize the
CNTFET fabrication technology as well. The role of
the Al/Ni films as “sacrificial” catalyst to stimulate
SWNT growth is evident from the AFM images of
Fig. 2 where the SWNTs always start to grow from a
Ni-cluster and extend on the SiO
2
. With topographic
AFM the SWNTs with a diameter of approximately
1 - 2 nm are clearly detectable on smooth thermally
grown SiO
2
. Examples of simple CNT network
structures are shown in Fig. 3.
2.2 Electrical CNTFET Device
Characteristics
Once the electrical connection with the S/D contacts
is established, the electrical characteristics of the
CNTFETs can be obtained as shown in Fig. 4. In a
CNTFET the electric field applied via the gate
electrode modulates the charge carrier density in the
nanotube and thus the current between the source (S)
and drain (D) electrodes. Figure 4 shows the
measured drain-current (Ids) as a function of the
gate voltage (Vgs) which is swept between positive
and negative values. Our fabricated devices are fully
Si
Metal Catalyst Deposition
SiO
2
Al (8 nm)
Ni (1 nm)
Si
Metal Catalyst Deposition
SiO
2
Si
Metal Catalyst Deposition
SiO
2
Al (8 nm)
Ni (1 nm)
Si
SiO
2
AlxOy
Pd
Pd
Pd S/D Contacts (Lift-Off)
Si
SiO
2
Si
SiO
2
AlxOy
Pd
Pd
Pd S/D Contacts (Lift-Off)
(Gate)
(S) (D)
Si
SiO
2
AlxOy
Pd
Pd
Pd S/D Contacts (Lift-Off)
Si
SiO
2
Si
SiO
2
AlxOy
Pd
Pd
Pd S/D Contacts (Lift-Off)
(Gate)
(S) (D)
SWNT
Cluster Formation + CVD
Ni clusters
Si
SiO
2
AlxOy
SWNT
Cluster Formation + CVD
Ni clusters
Si
SiO
2
SiO
2
AlxOy
Si
Metal Catalyst Deposition
SiO
2
Al (8 nm)
Ni (1 nm)
Si
Metal Catalyst Deposition
SiO
2
Si
Metal Catalyst Deposition
SiO
2
Al (8 nm)
Ni (1 nm)
Si
SiO
2
AlxOy
Pd
Pd
Pd S/D Contacts (Lift-Off)
Si
SiO
2
Si
SiO
2
AlxOy
Pd
Pd
Pd S/D Contacts (Lift-Off)
(Gate)
(S) (D)
Si
SiO
2
AlxOy
Pd
Pd
Pd S/D Contacts (Lift-Off)
Si
SiO
2
Si
SiO
2
AlxOy
Pd
Pd
Pd S/D Contacts (Lift-Off)
(Gate)
(S) (D)
SWNT
Cluster Formation + CVD
Ni clusters
Si
SiO
2
AlxOy
SWNT
Cluster Formation + CVD
Ni clusters
Si
SiO
2
SiO
2
AlxOy
Figure 1: Process flow of CNTFET and CNTFES
fabrication based on CVD with sacrificial catalyst.
nmnm
Figure 2: AFM scan of SWNTs with a diameter o
approximately 1 to 2 nm on test structure (left). Top view
of CNTFET/CNTFES with CNT connecting source and
drain electrodes (right).
Figure 3: Example of CNT network structures with cross-
over points.
BIODEVICES 2008 - International Conference on Biomedical Electronics and Devices
100