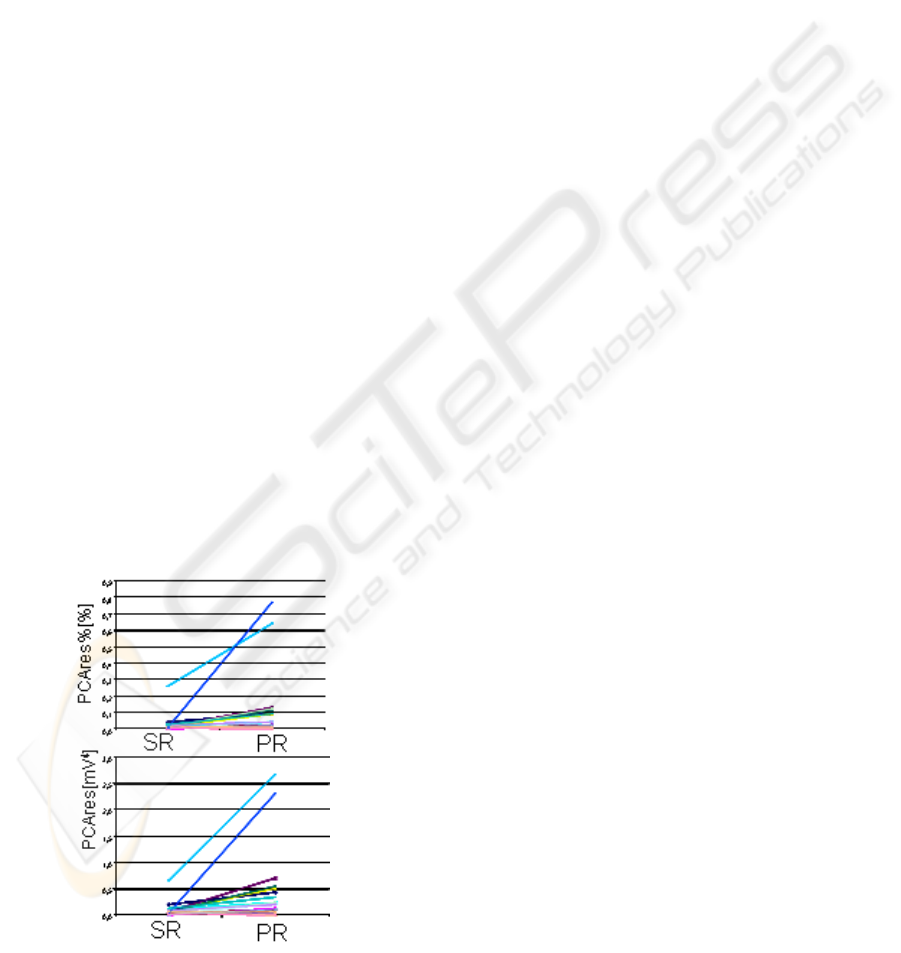
factor loading is the percent of variance in that
variable explained by that PC (i.e the degree of
correlation between the original data and the first
principal component expressed in percentage).
In addition, in order to estimate to which extent
each lead contributes to the first principal
component, factor loadings have been calculated.
Analogous to Pearson's coefficient, the squared
factor loading is the percent of variance in that
variable explained by that PC (i.e the degree of
correlation between the original data and the first
principal component expressed in percentage).
3 RESULTS
Figure 2 shows the 32 P-wave templates and the
results of the PCA for one patient.
Table 1 summarizes the results obtained by the
PCA parameters. The number of principal
components according to the latent root criterion
ranges between 2 and 3 (2,56±0,51) during
spontaneous rhythm and between 2 and 4 during
pacing (2,67±0,69, p=0,6).
PCA parameters related to the three largest PC
(PCA
1
[%], PCA
2
[%] and PCA
3
[%]), that describe
the dipolar component of the P-wave, did not
significantly differ during spontaneous and paced
rhythm (table 1, Wilcoxon test for paired data). The
not dipolar component (figure 3) as defined by both
PCAres and PCAres% during spontaneous rhythm
were significantly lower than during pacing
(PCAres%: 0,03±0,06 vs 0,12±0,21, p=0,001;
PCAres[mV
4
]: 0,10±0,14 vs 0,49±0,73, p=0,001).
Figure 3: Notdipolar components as defined by both
PCAres and PCAres% during spontaneous rhythm and
during pacing.
Factor analysis showed that on average all leads
contributes to the first principal components. Figure
4 shows the factor loadings averaged (in absolute
values) all over the population during spontaneous
rhythm and during pacing. Each lead but one in
spontaneous rhythm (lead A17) correlates with the
first principal component.
4 DISCUSSION
Analysis of the P-wave had been extensively
developed to extract parameters related to atrial
depolarization heterogeneities useful to recognize
patients with paroxymal AF or to predict the
development and the perpetuation of AF (Dilaveris
et al., 1998; Jordaens et al., 1998; Dilaveris et al.,
2001; darbar et al., 2002; Dilaveris et al., 2002).
However, the technical difficulties to acquire and
process the P-wave, had so far limited its clinical
use. Indeed, promising results have been obtained by
performing the PCA of the T-wave, in terms of
quantification of ventricular repolarization
inhomogeneity that may create an arrhythmogenic
ventricular substrate (De Ambroggi et al., 1997;
Acar et al, 1999; Malik et al., 2000; Kesek et al.,
2004).
We hereby used an 32-lead ECG acquisition
system particularly suitable for P-wave analysis,
having 24 bit resolution and being DC-coupled. We
performed the PCA of the P-wave in patients prone
to AF. PCA has been applied to the average P-wave
extracted in any of the 32 leads.
For each patient we extracted the same PCA
parameters employed for the T-wave (Kesek et al.,
2004; Acar et al, 1999). As for the T-wave, the PCA
parameters related to the first three PCs are
associated to the dipolar component of the P-wave,
while the remaining PCs (form the 4
th
to the 32
nd
)
are associated with the not dipolar component of the
P-wave.
To our knowledge this is the first time the PCA
is performed on the P-wave, thus physiological
interpretation and critical discussion can be related
only to previous experimental evidences of
ventricular conduction disturbance (PCA of the T-
wave ) (Kesek et al., 2004; Acar et al, 1999).
The first important result is that pacing provokes
a significant increase of the not dipolar components
of the P-wave. Thus atrial pacing changes the atrial
activation, disturbing the normal atrial
depolarization process and generating additional
paths not explainable by a single P-vector. Such a
PRINCIPAL COMPONENT ANALYSIS OF THE P-WAVE - Quantification of Not-Dipolar Components of Atrial
Depolarization
555