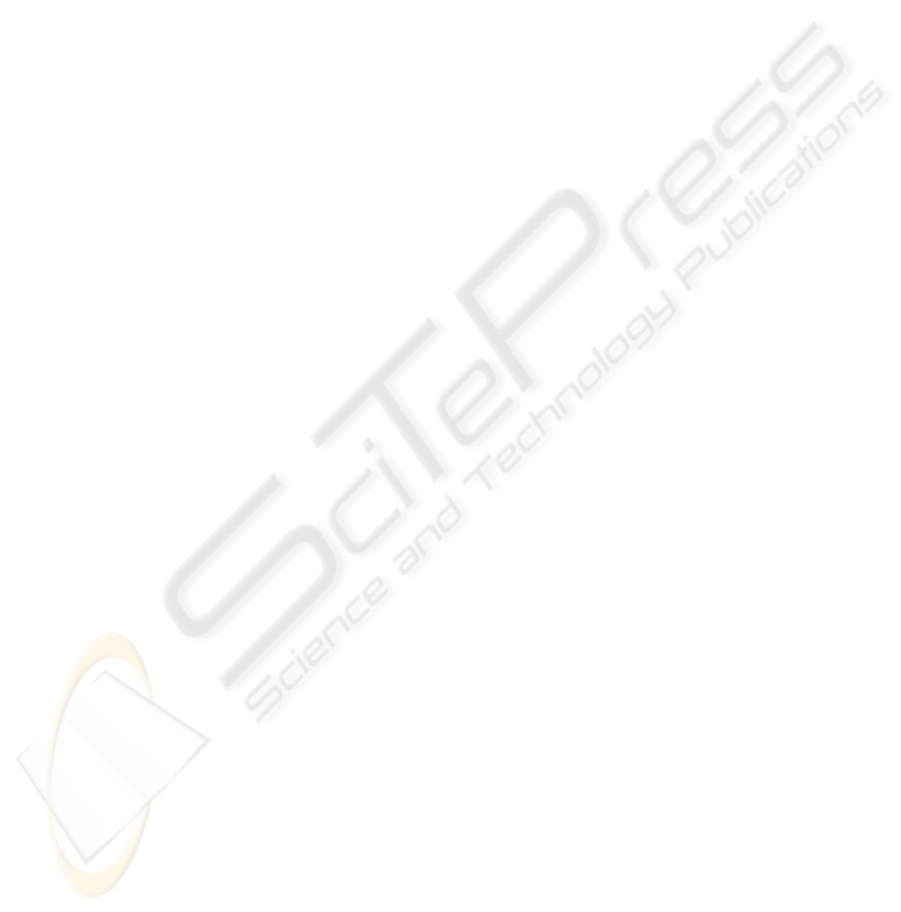
ever for short pulses (10-15ms) it is significantly
lower then for the traditional stimulation at 50Hz (fig.
2). In case of the modulated HFS, the RCD is over
five times lower in comparison to the results of the
harmonic persistent stimulation. This observation can
be explained on the basis of the DICR model, because
the modulated sinusoidal stimulation ensures the re-
fractory period for the DHPR receptor.
5.3 Discussion
Presented myofibril model reflects effects of Ca
2+
re-
lease from SR as a result of sarcolemma depolariza-
tion. It does not take into consideration the proper-
ties of the sarcolemma and other tissues which are
stimulated during NMES. Thereby, the effect of di-
rect influence of a transcutaneous stimulus on DHPR
receptor can not be clearly established. It could be ex-
plained only on the basis of in vivo experiment results
and on a muscle model reflecting myofibril proper-
ties, muscle fibres recruitation during stimulation and
electrical properties of the skin and other tissues com-
bined.
Modulated HFS trains seem to do better than the
traditional stimulation programs, however the influ-
ence of such a stimulation on the fibre degeneration
process should be investigated. Although the ampli-
tude of repolarization pulses during HFS stimulation
are 50% lower as compared to the short-pulses stim-
ulation, the mean stimulation current is significantly
higher (Bennie et al., 2002). In comparison with the
wide-pulse stimulation the modulated HFS seems to
be less painful due to the lower tissue impedance at
a higher frequency. It should be mentioned that the
presented model and results can be useful to evalu-
ate stimulation programs under the hypothesis that the
transcutaneus stimulation can trigger the DICR effect.
ACKNOWLEDGEMENTS
The work was partially supported by the Polish
Ministry of Education and Science, project no.
1445/T11/2004/27
REFERENCES
Bakker, A. J., Head, S. I., and Stephenson, D. G. (1996).
Measurement of membrane potential and myoplasmic
[ca2+] in developing rat myotubes at rest and in re-
sponse to stimulation. Cell Calcium, 19(5):409 – 418.
Benders, A. A., Oosterhof, A., Wevers, R. A., and
Veerkamp, J. H. (1997). Excitation-contraction cou-
pling of cultured human skeletal muscle cells and the
relation between basal cytosolic ca2+ and excitability.
Cell Calcium, 21(1):81 – 91.
Bennie, S. D., Petrofsky, J. S., Nisperos, J., Tsurudome, M.,
and Laymon, M. (2002). Toward the optimal wave-
form for electrical stimulation of human muscle. Eur
J Appl Physiol, 88(1-2):13 – 19.
Bottinelli, R. and Reggiani, C. (2000). Human skele-
tal muscle fibres: molecular and functional diversity.
Progress in Biophysics & Molecular Biology, 73:195
– 262.
Chou, L.-W., Ding, J., Wexler, A. S., and Binder-Macleod,
S. A. (2005). Predicting optimal electrical stimulation
for repetitive human muscle activation. J Electromyo-
graphy Kinesiology, 15:300–309.
Delbono, O. and Meissner, G. (1996). Sarcoplasmic reticu-
lum ca2+ release in rat slow- and fast-twitch muscles.
J. Membr. Biol., 151(2):123 – 130.
Ding, J., Wexler, A. S., and Binder-Macleod, S. A. (2003).
A mathematical model for fatigue minimization dur-
ing functional electrical stimulation. Journal of Elec-
tromyography and Kinesiology, 13:575–588.
Gissel, H. (2000). Ca2+ accumulation and cell damage in
skeletal muscle during low frequency stimulation. Eur
J Appl Physiol, 83(2-3):175 – 180.
Glukhovski, A., Adam, D., Amitzur, G., and Sideman, S.
(1998). Mechanism of ca2+ release from the sar-
coplasmic reticulum: a computer model. Ann Biomed
Eng, 26:213–229.
Green, R. and Laycock, J. (1990). Objective methods for
evaluation interferential therapy in the treatment of in-
continence. IEEE Trans Biomed Eng, 37(6):615–623.
Kostyukov, A. I., Hellstrom, F., Korchak, O. E.,
Radovanovic, S., Ljubisavljevic, M., Windhorst, U.,
and Johansson, H. (2000). Fatigue effects in the cat
gastrocnemius during frequency-modulated efferent
stimulation. Neuroscience, 97(4):789 – 799.
Mourselas, N. and Granat, M. H. (1998). Evaluation of pat-
terned stimulation for use in surface electrical stim-
ulation systems. Medical Engineering and Physics,
20:319–324.
Otazu, G. H., Futami, R., and Hoshimiya, N. (2001). A
muscle activation model of variable stimulation fre-
quency response and stimulation history, based on
positive feedback in calcium dynamics. Biol Cybern,
84(3):193 – 206.
Riener, R. and Quintern, J. (1997). A physiologically based
model of muscle activation verified by electrical stim-
ulation. Biochemistry and Bioenergetics, 43:257–264.
Wallinga, W., Meijer, S. L., Alberink, M. J., Vliek, M.,
Wienk, E. D., and Ypey, D. L. (1999). Modelling ac-
tion potentials and membrane currents of mammalian
skeletal muscle fibres in coherence with potassium
concentration changes in the t-tubular system. Eur
Biophys J, 28(4):317 – 329.
Westerblad, H., Bruton, J. D., Allen, D. G., and Lannergren,
J. (2000). Functional significance of ca2+ in long-
lasting fatigue of skeletal muscle. Eur J Appl Physiol,
83(2-3):166 – 174.
BIOPHYSICAL MODEL OF A MUSCLE FATIGUE PROCESS INVOLVING Ca2+ RELEASE DYNAMICS UPON
THE HIGH FREQUENCY ELECTRICAL STIMULATION
57