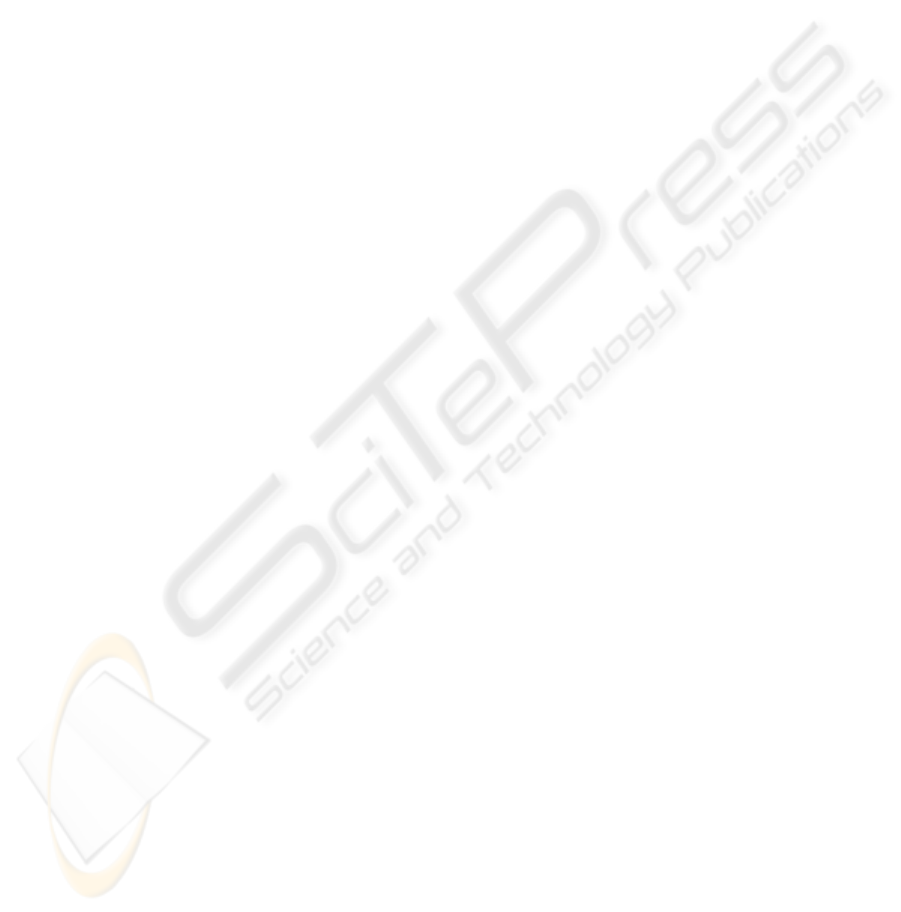
ventricular activation sequence, our findings suggest
that within the first 20 ms of the ventricular
activation paraseptal parts of the right ventricle
could contribute to the observed differences in
CMFM topology. In contrast, the map topology
differences at the end of the QRS could reflect the
influence of regional LV wall hypertrophy and
myocardial fibrosis on the electrophysiological
myocardial properties, especially if the propagation
wave front turns from apical to posterior basal LV.
These findings are consistent with those from
invasive electrophysiological and morphological LV
studies (Schumacher, 2005). Myocardial scarring
and its electrophysiological consequences like
slowed and fragmented intraventricular conduction
also contributed to the specific magneto-
physiological HCM phenotype.
Changes in repolarization in HCM patients were
also found in ECG studies (Barletta, 2005). The
most common abnormalities are related to the ST-
segment and the T-wave. This is in consistence with
our findings of differences in KL entropy values at
the STT interval. They probably emanate from
myocardial disarray, fibrosis and small vessel
disease leading to scarred myocardium due to
regional ischemia (Basso, 2000). HCM does not
affect the ventricles uniformly; it is likely that there
are areas of diseased myocardium with
abnormalities in conduction and refractoriness and
heterogeneity of refractoriness, especially related to
distal hypertrophy with craniocaudal asymmetry.
Compared with KL measurements, we also
found significant regional deviations of magnetic
field strengths during depolarization period (QRS),
especially in the superior (sensor position B3) and
inferior (sensor position F3) part of the mapped area.
However, the overall classification rate using only
these parameters was lower compared to the KL
based set. Specificity was comparable with KL
method but sensitivity was substantially lower. A
possible explanation for the lower classification rate
could be that regional parameters are more sensitive
to measurement conditions, especially to the position
of the patient’s heart relative to the measurement
system. Even with a presumed constant distance
between sensors and thorax surface, the variations in
patients’ anatomy result in different heart-sensor
distances. Automatic adjustments to solve this
problem are under investigation (Burghoff, 2000).
In contrast to the lower efficacy of the mean
values of magnetic field strength approach, the
classification rate improved adding a regional
parameter to the KL features. Since the
crossvalidation did not differ from the original
results the improvement in classification is due to a
higher information content of the combined
parameter set.
Discrimination of Obstructive from non
Obstructive Forms of HCM. In order to find a
discriminant function for separation of HCM
subforms (HOCM vs. HNCM), we applied the same
approach but now using the HOCM group maps as
the reference for KL entropy calculation. As shown
by high DI values, KL based topology differed only
in a short time interval within the second part of
depolarization process (57-77ms). The analysis of
regional magnetic field differences revealed that
most significant differences between these two
HCM subforms exist in the inferior part of the
mapped area (sensor position F5). HOCM is
characterized by a predominantly septal
hypertrophy, which leads to chronic obstruction of
the left ventricular outflow tract and consecutively to
an increase in wall stress, myocardial ischemia,
increased cell death and fibrosis.
Using gadolinium contrast-enhanced MRI,
Choudhury et al. found in asymptomatic or mildly
symptomatic patients with HCM that the extent of
scar increased significantly in relation to wall
thickness on a regional basis. The
electrophysiological consequences are regional
prolongation of the bipolar endocardial potentials
and the occurrence of fractionated and split
potentials, which directly point to an underlying
inhomogeneity of the myocardial excitation with a
shift to earlier activation of the lateral LV wall due
to septal conduction delay. This probably led to the
observed deviation in CMFM map topology between
HOCM and HNCM patients in the second part of the
QRS interval, which could be quantified by using
the KL entropy method. The alterations of regional
electrophysiological properties at hypertrophic
septal areas are responsible for the observed changes
in the inferior mapped area.
Intended to detect HCM subforms, KL entropy
measures were superior to the analysis of regional
map differences. But, adding a regional parameter
QRSA3 to KL entropy parameters, the classification
result improved to 97 % with a sensitivity of 100 %
for HNCM and a specificity of 94.7 % for HOCM.
Feasibility of the Approach and Conclusions. The
correct classification of 5 HOCM and HNCM
patients out of 22 family members, in which the
diagnosis was confirmed by genetic testing, showed
in a prospective part of the study the feasibility of
the presented diagnostic algorithm. Our results give
CARDIAC MAGNETIC FIELD MAP TOPOLOGY QUANTIFIED BY KULLBACK-LEIBLER ENTROPY IDENTIFIES
PATIENTS WITH HYPERTROPHIC CARDIOMYOPATHY
451