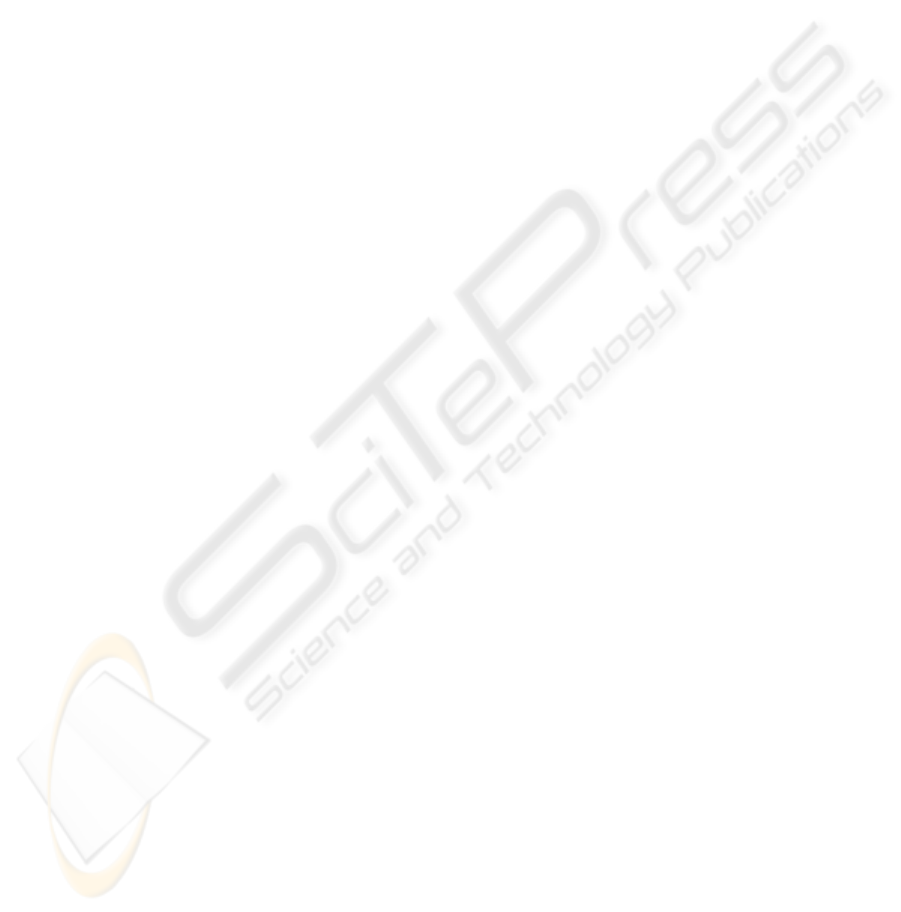
7 CONCLUSIONS
This work provides the data measured along several
years of experimental research in the 5 GHz band,
including electromagnetic characterisation of
different building materials, indoor radio channels
analysis, as well as non deterministic effects as those
introduced by people moving or by furniture within
an static environment.
The complex permittivity values provided for
two orthogonal polarisations and three walls of
different kinds could be used in simulation tools to
define indoor environments constructed by typical
building materials.
A power decay law as a function of distance
between transmitter and receiver is also provided at
different environments in both LoS and OLoS
conditions. Fast variations due to multipath
propagation appear, added to this main tendency.
These variations follow a Weibull distribution.
Broad band radio channel parameters, as time
delay and coherence bandwidth, are presented in this
work.
Moreover, non-deterministic effects have been
also measured and reported. These added
contributions, which are due to the presence of
furniture or to people in motion within the
environment, could be modelled as a random
contribution added to the deterministic one.
Results show that the presence of furniture and
decorative objects deteriorates the performance of
the radio channel. The attenuation with distance
grows, as well as new fading events appear, as the
presence of new elements in the environment
produces reflection of waves and scattering. When
furnishing a room, coherence bandwidth is reduced
by 29% at 0.7 and 25% at 0.9 correlation levels,
probably due to the new multipath components that
are received as a result of the presence of more
scatterers in the environment.
The movement of people seems to have small
influence in long-time average response of the
channel (median received power), although it could
be strongly influent in short time periods. Besides,
punctual highly constructive or deeply destructive
interference can occur when people acts in the
environment.
This information could be helpful for network
designers to better predict the network performance
and to prevent possible external non authorised
access or isolation problems, as the more exact the
planning is, the less coverage problems could
appear.
ACKNOWLEDGEMENTS
This work has been supported by Xunta de Galicia,
Project Ref. PGIDIT05TAM32201PR.
REFERENCES
Burnside, W.D., Burgener, K.W., January 1983. “High
frequency scattering by a thin lossless dielectric slab”,
IEEE Transactions on Antennas and Propagation, vol.
Ap-31, no. 1, pp. 104-110.
Cuiñas, I., Sánchez, M.G., Pellicer, A.M., 1999.
“Integrated automated system for microwave
reflection coefficient measurement”, IAIA Third
International Conference on Industrial Automation,
Montreal (Canada).
Cuiñas, I., García Sánchez, M., March 2001. "Measuring,
Modeling and Characterizing of Indoor Radio Channel
at 5.8 GHz", IEEE Transactions on Vehicular
Technology, vol. 50, no. 2, pp. 526-535.
Cuiñas, I., Martínez, D., García Sánchez, M., Vázquez
Alejos, A., April 2007. “Modelling and measuring
reflection due to flat dielectric surfaces at 5.8 GHz”,
IEEE Transactions on Antennas and Propagation, vol.
55, no. 4, pp. 1139-1147.
Dersch, U., Zollinger, E., November 1994. "Propagation
mechanisms in microcell and indoor environments",
IEEE Transactions on Vehicular Technology, vol. 43,
no. 4.
Dossi, L., Tartara, G., Tallone, F., April 1996. "Statistical
Analysis of Measured Impulse Response Functions of
2.0 GHz Indoor Radio Channels", IEEE Journal on
Selected Areas in Communications, vol. 14, no. 3,
pp.405-410.
Dutta-Roy, A., December 1999. "Networks for Homes",
IEEE Spectrum, pp.26-33.
Hashemi, H., McGuire, M., Vlasschaert, T., Tholl, D.,
August 1994. "Measurements and modelling of
temporal variations of the indoor propagation
channel", IEEE Transactions on Vehicular
Technologies, col. 43, no. 3, pp. 733-737.
IEEE Standard 802.16a-2003, IEEE Standard for Local
and metropolitan area networks — Part 16: Air
Interface for Fixed Broadband Wireless Access
Systems — Amendment 2: Medium Access Control
Modifications and Additional Physical Layer
Specifications for 2-11 GHz.
Sagnard, F., El Zein, G., October 2005. “In situ
characterization of building materials for propagation
modelling: frequency and time responses”, IEEE
Transactions on Antennas and Propagation, vol. 53,
no. 10, pp. 3166-3173.
Sagnard, F., Bentabet, F., Vignat, C., June 2005, “In situ
measurements of the complex permittivity of materials
using reflection ellipsometry in the microwave band:
experiments (Part II)”, IEEE Transactions on
Instrumentation and Measurement, vol. 54, no. 3, pp.
1274-1282.
WINSYS 2008 - International Conference on Wireless Information Networks and Systems
134