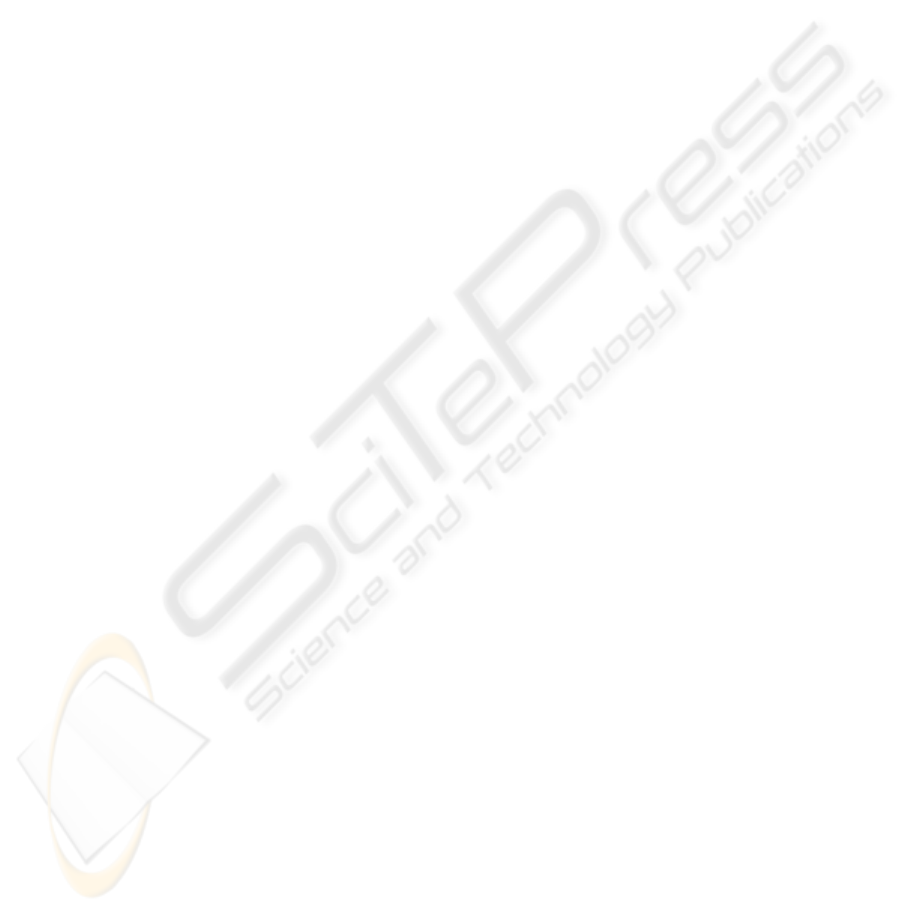
VIRTUAL FREQUENCY REUSE TO INCREASE CAPACITY OF
OFDM SYSTEMS
Seung-Moo Cho and Tae-Jin Lee
School of Information and Communication Engineering, Sungkyunkwan University, Suwon, 440-746, South Korea
Keywords:
Frequency reuse, fractional frequency reuse (FFR), orthogonal frequency division multiplexing (OFDM), re-
source management.
Abstract:
This paper presents a novel frequency reuse scheme that reduces the effects of co-channel interference and in-
creases the capacity of orthogonal frequency division multiplexing (OFDM) systems. To increase the capacity
of a system, the frequency reuse factor should be close to 1. In general, reduction of co-channel interference
(CCI) is achieved at the cost of cell capacity. Our virtual frequency reuse (VFR) targets to mitigate such
tradeoff. In VFR, the type of a cell is determined by the order of sub-channel assignment. And users in a
cell are assigned sub-carriers among sub-channels by a specific regulation. Probabilistic interference analysis
and simulation results show that the proposed virtual frequency reuse improves the performance of an OFDM
system for both uniform and non-uniform distributions of traffic load.
1 INTRODUCTION
To support the emergence of new wireless appli-
cations and the proliferation of multimedia ser-
vices, broadband wireless access (BWA) has been re-
searched (A. Jamalipour and Yamazato, 2005). Due
to limited spectral resources, next-generation wireless
networks require some techniques to utilize frequency
spectrum efficiently. The orthogonal frequency divi-
sion multiplexing (OFDM) is considered as one of the
best solutions to satisfy this requirement (M. Sternad
and Brunstrom, 2007), (M. Bohge and Meyer, 2007).
In OFDM, the parallel transmission of data symbols
deceases the effect of intersymbol interference (ISI),
which is appropriate for BWA.
To increase the spectral efficiency in OFDM, spec-
tral resource management is necessary. Many chan-
nel assignment techniques are proposed to manage
spectral resources efficiently in OFDM systems. Ba-
sically, channel assignment techniques are classified
into fixed channel assignment (FCA) and dynamic
channel assignment (DCA). FCA assigns a set of
channels to each cell permanently. So, FCA is simple
and shows reasonable performance. However, if a cell
has high traffic load and the other cells have lowtraffic
load, the spectral resources may not be managed effi-
ciently in FCA. To improvethe shortcomings of FCA,
DCA is proposed (S. Anand and Sivarajan, 2003). In
DCA, channels may be assigned to cells during a spe-
cific time duration and the assignment changes dy-
namically. It reflects the traffic condition of each cell
and manages the spectral resources efficiently. Since
DCA causes unexpected interference to neighboring
cells, interference avoidance algorithms are required.
Combining FCA and DCA, borrowing channel as-
signment (BCA) is proposed (Jiang and Rappaport,
1996). A cell in high traffic condition can borrow
channels from neighboring cells to accept incoming
calls. BCA improves the performance of FCA and
reduces the overhead caused by exchange of channel
assignment in DCA.
In wireless cellular systems, the frequency reuse is
employed to reduce the effects of co-channel interfer-
ence (CCI) and to increase the capacity of a system.
The reuse factor should be close to 1 to increase the
system capacity. But, the reduction of CCI is achieved
at the cost of cell capacity. To mitigate such tradeoff,
some techniques such as reuse partitioning and frac-
tional frequency reuse (FFR) have been studied (Chu
and Rappaport, 1997), (Forum, 2006). Reuse parti-
tioning uses multiple reuse factors. Overlaid cells are
implemented to reduce the CCI in reuse partitioning.
FFR has constraints on a usable set of channels in
cells to balance the tradeoff between the cell capac-
ity and the interference.
In this paper, we propose a new virtual frequency
135
Cho S. and Lee T. (2008).
VIRTUAL FREQUENCY REUSE TO INCREASE CAPACITY OF OFDM SYSTEMS.
In Proceedings of the International Conference on Wireless Information Networks and Systems, pages 135-139
DOI: 10.5220/0002021601350139
Copyright
c
SciTePress