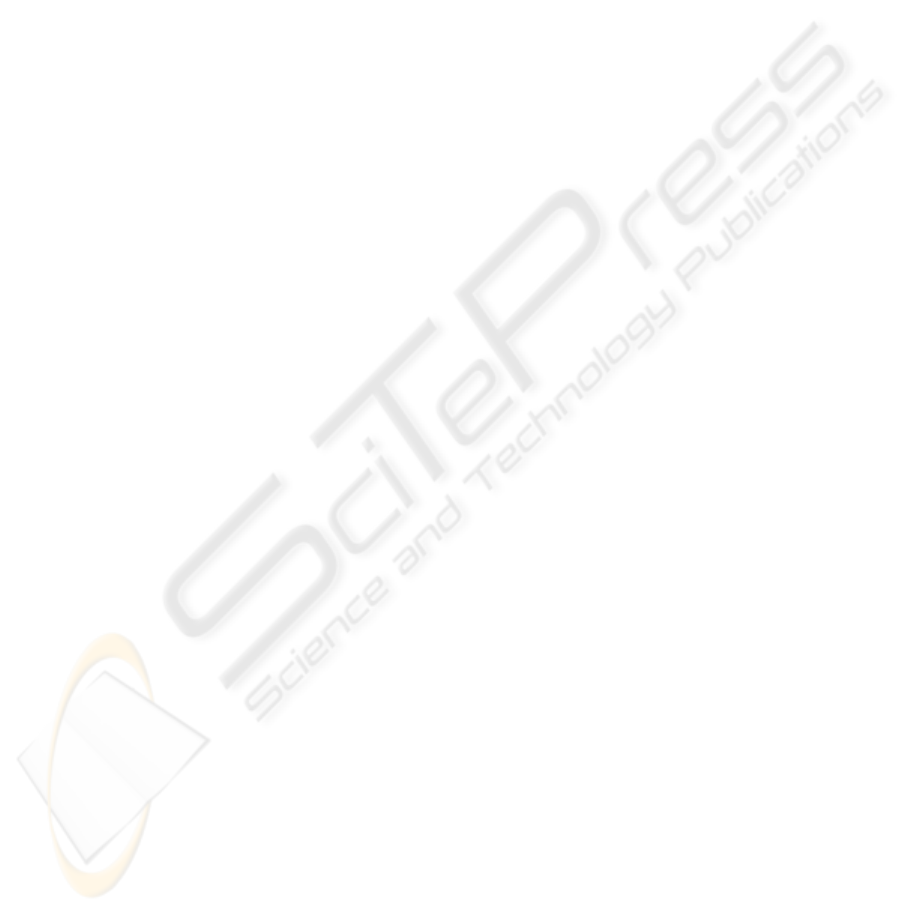
beta, mu and nu. The precursors (mu and nu), when
exposed to alkali conditions, are modified into kappa
and iota, respectively, through formation of the 3,6-
anhydro-galactose bridge (Rudolph, 2000).
Infrared (IR) spectroscopy was, until recently the
most frequently used vibrational technique for the
study of the chemical composition of phycocolloids.
This technique presents two main advantages: it
requires minute amounts of sample (milligrams),
and it is non-aggressive method with reliable
accuracy (Givernaud-Mouradi, 1992; Pereira et
al.,2003). However, conventional IR spectroscopy
requires laborious procedures to obtain spectra with
a good signal/noise ratio (Chopin and Whalen,
1993). This limitation was overcome with the
development of interferometric IR techniques
(associated with the Fourier transform algorithm),
known as FTIR spectroscopy (Fourier Transform
IR). More recently, Pereira and collaborators had
used a technique of analysis on the basis of FTIR-
ATR (from Attenuated Total Reflectance)
spectroscopy, allowing for the determination of the
composition of the different phycocolloids from
dried ground seaweed, without having to prepare
tablets of KBr (Pereira, 2006; Pereira and Mesquita,
2004).
In contrast to FTIR, the application of
conventional Raman spectroscopy was limited until
recently, due to need for an incident visible laser in
dispersive spectrometers: the visible laser light often
excites electronic transitions in biochemical
samples, which can lead to either sample
degradation or strong background signal from
unwanted laser-induced fluorescence. The use of
Nd:YAG lasers operating at 1064 nm (far from the
visible region) in interferometric spectrometers has
been generalized to decrease the fluorescence level
and avoid sample degradation. The modern FT-
Raman spectrometers have been used to produce
good quality Raman spectra from seaweed samples.
(Matsuhiro, 1996; Pereira et al., 2003).
In this work, a combined FTIR-ATR and FT-
Raman spectroscopy study were used to identify the
colloid produced by one of the principal source of
carrageenans, the red algae Chondrus crispus. Since
the analysis of ground seaweed samples required
minimal treatment (the seaweeds are simply dried
and ground), the determined composition represents,
as accurately as possible, the natural colloid
composition.
2 MATERIALS AND METHODS
2.1 Algal Material and Standard
Samples of Phycocolloids
Specimens of red algae (Rhodophyceae) Chondrus
crispus are collected in the central zone of the
western coast of Portugal (wild specimens) and other
are cultivated in Canada (lambda strain). Standard
samples were obtained from Sigma (type IV, C-
3889) and CP Kelco (pure lambda-carrageenan).
The sample composition and purity were
controlled by NMR.
2.2 Preparation of Ground Seaweed
Samples for FTIR-ATR and
FT-Raman
The seaweed samples were rinsed in distilled
freshwater to eliminate salt and debris from the
thallus surface and dried to constant weight at 60 ºC.
The dried seaweeds were finely ground in order to
render the samples uniform. For FTIR analysis the
samples do not need additional treatment. The
analysis by FT-Raman requires that these are
without pigmentation. The lack of pigmentation can
be achieved by sun drying (process used by
collectors/producers of commercial seaweeds) or by
pigment elimination in the laboratory by the addition
of acetone/methanol moisture (V/V) or by the
addition of calcium hypochlorite solution (4%, 30/60
s, 4 ºC) (Pereira, 2004).
2.3 Phycocolloid Extraction
Before phycocolloid extraction, the ground dry
material was rehydrated and pre-treated in acetone
followed by ethanol to eliminate the organosoluble
fraction (Zinoun and Cosson, 1996).
For extraction of the native phycocolloid, the
seaweed samples were placed in distilled water (50
ml/g), pH 7 at 85º C for 3 h. For an alkaline-
extraction (resembling the industrial method), the
samples were placed in a solution (150 mL/g) of
NaOH (1 M) at 80-85 ºC for 3-4 h according to
Pereira and Mesquita (2004), and neutralised to pH
6-8 with HCl (0.3 M).
The solutions were hot filtered, twice, under
vacuum, through cloth and glass fibre filter. The
extract was evaporated under vacuum to one-third of
the initial volume. The carrageenan was precipitated
by adding the warm solution to twice its volume of
ethanol (96 %).
BIODEVICES 2009 - International Conference on Biomedical Electronics and Devices
132