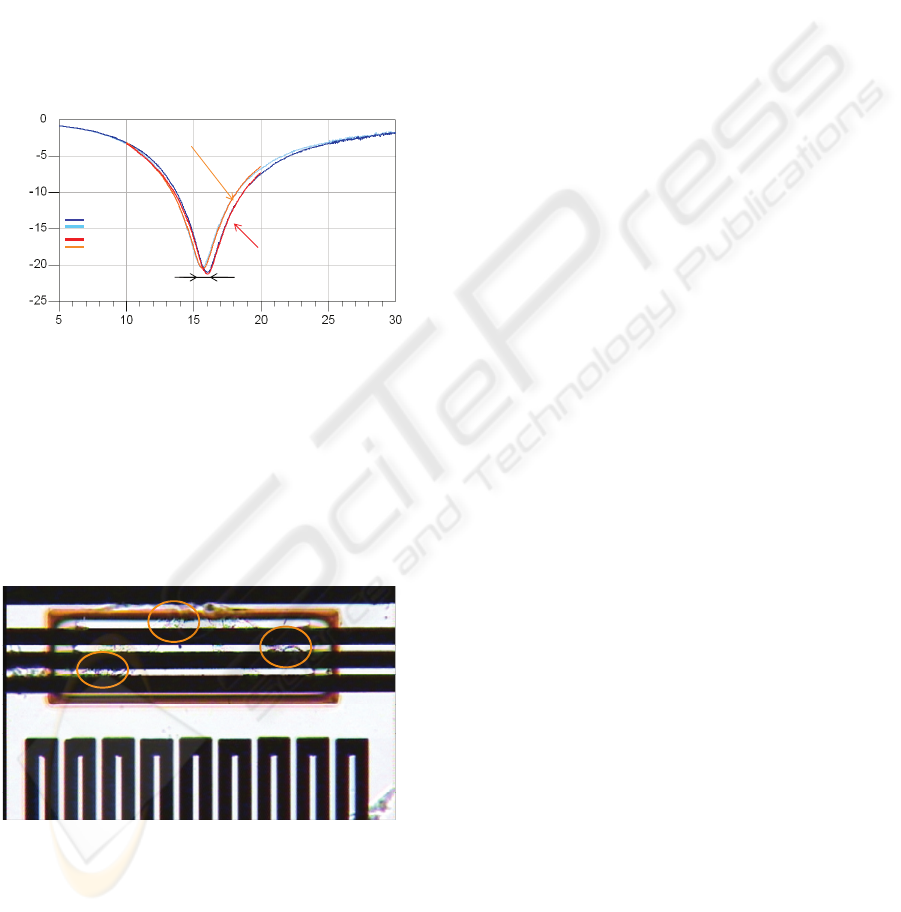
3 EXPERIMENTAL RESULTS
All characterizations are performed using classical
microwave measurement techniques with on wafer
probing; as it allows a quick and a successive sensor
measurements.
First, for each sensor, the transmitted microwave
signal attenuation across the unloaded resonator is
recorded using a calibrated vector network analyser.
Then cell culture is performed; at the end, loaded
sensors with fixed cells are measured following the
same procedure than before cell growth. Hence, the
induced resonator frequency shift value, related to
the cells electrical properties can be extracted.
Frequency (GHz)
S
21
(dB)
Unloaded sensor
EM simulation
Measurement
Sensor after cell growth
370MHz
Figure 7: Biosensor measured response before and after
the cell growth and simulated one.
Figure 7 shows results of experimentations with
glial-cells-derived tumour glioblastoma coming
from human nervous system cells. Used biosensors
initially resonate at 16 GHz and shift down to 15.63
GHz when it is loaded with only 8 glial-cells (figure
8).
Figure 8: Photograph of the sensor after the cell growth.
Fullwave simulations, based on finite element
method (HFSS from ANSOFT), are then used to
extract individual cell electrical properties. Cells EM
modelling is done assuming that they are
homogenous, source-free and linear dielectric
volume. Hence, on a narrow frequency bandwidth
around the sensor resonant frequency, cell global
permittivity and conductivity can be extracted with a
good accuracy by fitting simulations data with
measured one, as shown on figure 7.
Hence in the case of analysed glial-cells, we
have obtained an effective permittivity value of 36 ±
1 while global conductivity has been estimated
0.100 ± 0.003 S/m at 16 GHz and 20°C. These
results agree very well with previous analysis done
with ficoll media (Dalmay et al., 2008) and can be
compared to the effective permittivity of pure water
which is closed to 45 at 20°C. Other
characterizations are currently done with other
cellular types, to demonstrate that it is possible with
this approach to discriminate between different cell
types.
4 CONCLUSIONS
An original label free bio-sensing approach for
cellular analysis at radio frequencies has been
demonstrated. Thanks to their sub millimetric size,
used sensors are able to work at the cell scale with a
very limited number of cells and can potentially be a
novel promising tool for cell discrimination. Further
work is ongoing to evaluate experimentally the
minimum number of cell analysis achievable and to
improve the sensor design and experimental process
for one single cell analysis.
REFERENCES
Young-Il Kim, Yunkwon Park, Hong Koo Baik, 2007.
“Development of LC resonator for label-free
biomolecule detection”, Sensors and Actuators A.
B. Blad, and B. Baldetorp, 1996. “Impedance spectra of
tumour tissue in comparison with normal tissue; a
possible clinical application for electrical impedance
tomography”, Physiol.Meas., vol. 17, pp. 105- 115.
T. W. Athey, M. A. Stuchly, S. S. Stuchly, 1982.
“Measurement of radio frequency permittivity of
biological tissues with an open-ended coaxial line :
Part I,” IEEE Trans. Microwave Theory Tech,. vol.
82, pp. 82-86.
N. Denef , L. Moreno-Hagelsieb, G. Laurent, R. Pampina,
B. Foultier, J. Remacle, D. Flandre, 2004. “RF
detection of DNA based on CMOS inductive and
capacitive sensors”, EUMW Conference Digest, pp.
669-672.
C. Dalmay, 2008. “Label free biosensors for human cell
characterization using radio and microwave
frequencies”, IEEE MTT-S International Microwave
Symposium Digest, IMS 2008.
BIODEVICES 2009 - International Conference on Biomedical Electronics and Devices
6