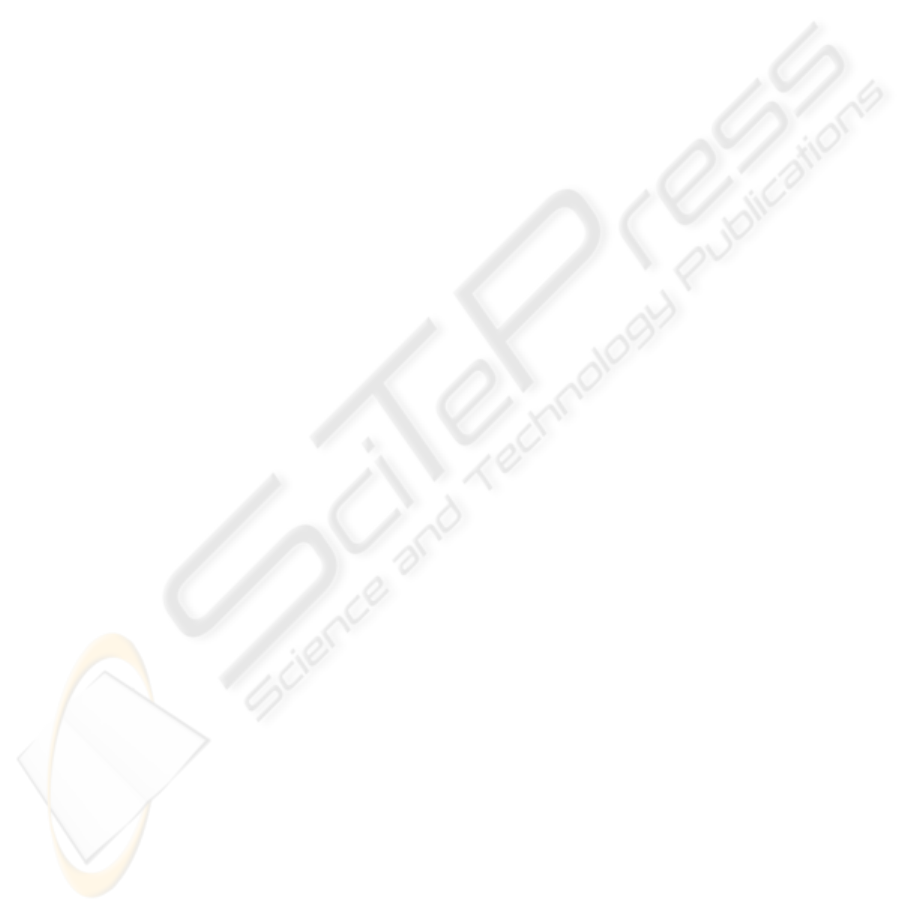
ACKNOWLEDGEMENTS
The authors would like to thank colleagues from the
Portuguese PET Consortium and the Crystal Clear
Collaboration for their suggestions and contribution.
This project is financed by AdI (Agência de
Inovação) and POSI (Operational Program for
Information Society), Portugal. The work of P.
Rodrigues and A. Trindade was supported by FCT
under grants SFRH/BPD/37233/2007 and
SFRH/BPD/37226/2007. The work of R. Bugalho,
B. Carriço, C. S. Ferreira, R. Moura, C. Ortigão and
J. F. Pinheiro was supported by AdI.
REFERENCES
Abreu, M. C., Aguiar, J. D., Almeida, F. G., Almeida, P.,
Bento, P., Carriço, B., Ferreira, M., Ferreira, N. C.,
Gonçalves, F., Leong, C., Lopes, F., Lousã, P.,
Martins, M. V., Matela, N., Mendes, P. R., Moura, R.,
Nobre, J., Oliveira, N., Ortigão, C., Peralta, L.,
Pereira, R., Rego, J., Ribeiro, R., Rodrigues, P.,
Sampaio, J., Santos, A. I., Silva, L., Silva, J. C., Sousa,
P., Teixeira, I. C., Teixeira, J. P., Trindade, A.,and
Varela, J. (2006). Design and evaluation of the Clear-
PEM scanner for positron emission mammography.
IEEE Trans. Nuc. Sci., 53:71–77.
Abreu, M. C., Amaral, P., Carriço, B., Ferreira, M.,
Moura, R., Ortigãoo, C., Rato, P., and Varela, J.
(2007). Characterization and quality control of
avalanche PhotoDiode arrays for the Clear–PEM
detector modules. Nucl. Instr. and Method.,
576(1):19–22.
Albuquerque, A., V. Bexiga, Bugalho, R., Carriço, B.,
Ferreira, C. S., Ferreira, M., Godinho, J. Gonçalves,
F., Leong, C., Lousã, P., Machado, P., Moura, R.,
Neves, P., Ortigão, C., Piedade, F., Pinheiro, J. F,
Rivetti, A., Rodrigues, P., Silva, J. C., Silva, M. M.,
Teixeira, I. C., Teixeira, J. P., Trindade, A., Varela, J.
(2008) Experimental characterization of the 192
channel Clear–PEM frontend ASIC for multi–pixel
APD readout. Sub. Nucl. Instr. And Method.
Albuquerque, E., Almeida, F. G., Almeida, P., Augusto,
S., Bexiga, V., Bugalho, R. Carmona, S. , Carriço, B.,
Ferreira, C. S., Ferreira, N. C., Ferreira, M., Godinho,
J., Gonçalves, F., Guerreiro, C., Leong, C., Lousã, P.,
Machado, P., Martins, M. V., Matela, N., Moura, R.,
Neves, P., Oliveira, N., Ortigão, C., Piedade, F.,
Pinheiro, J. F., Rego, J., Relvas, P., Rivetti, A.,
Rodrigues, P., Sá, D. N., Sampaio, J., Santos, A. I.,
Silva, M. M., Teixeira, I. C., Teixeira, J. P., Silva, J.
C., Trindade, A., Varela, J. Performance evaluation of
a highly integrated APD/ASIC double-readout
supermodule with 768 channels for Clear-PEM, In
2008 IEEE Nuclear Science Symposium Conference
Record.
Amaral, P., Carriço, B., Ferreira, M., Moura, R., Ortigão,
C., Rodrigues, P., Silva, J. C., Trindade, A., and
Varela, J. (2006). Performance and quality control of
Clear–PEM detector modules. Nucl. Instr. and
Method. in press.
Albuquerque, E., Bento, P., Leong, C., Gonçalves, F.,
Nobre, J., Rego, J., Relvas, P., Lousã, P., Rodrigues,
P., Teixeira, I. C., Teixeira, J. P., Silva, L., Silva, M.
M., Trindade, A., and Varela, J. (2006). The Clear-
PEM electronics system. IEEE Trans. Nuc. Sci.,
53(5):2704–2711.
Crespo, P., Kapusta, M., Pawelke, J., Moszyński, M., and
Enghardt, W. (2004). First in–beam PET imaging with
LSO/APD array detectors. IEEE Trans. Nuc. Sci.,
51(5):2654–2661.
Kapusta, M., Crespo, P., Wolski, D., Moszyński, M., and
Enghardt, W. (2003). Hamamatsu S8550 arrays for
high–resolution scintillator matrices readout. Nucl.
Instr. and Method., A504:139–142.
P. Lecoq and J. Varela, Clear-PEM, a dedicated PET
camera for mammography. Nucl. Instrum. Meth. vol
A 486, pp.1-6, 2002.
Melcher, C. L. and Schweitzer, J. S. (1992). Cerium–
doped lutetium oxyorthosilicate: a fast, efficient new
scintillator. IEEE Trans. Nuc. Sci., 39(4):502–505.
Moses, W. W. (2004). Positron emission mammography
imaging. Nucl. Instr. and Method., A525(1–2).
Mosset, J. B. (2006). Developpement d’un module de
d´etection phoswich LSO%LuYAP pour le prototype
de camera `a positrons ClearPET. PhD thesis, Faculte
des Sciences de base de l´Ecole Polytechnique
Federale de Lausanne.
Rodrigues, P.,. (2007). Study and Development of the
Clear-PEM Trigger and Data Acquisition System
ClearPET. PhD thesis, Instituto Superior Técnico,
Universidade Técnica de Lisboa.
Spanoudaki, V., McElroy, D. P., Zell, K., and Ziegler, S. I.
(2005). Effect of temperature on the stability and
performance of an LSO–APD PET scanner. In 2005
IEEE Nuclear Science Symposium Conference
Record, pages 2785–2789.
Shao, Y., Silverman, R. W., Farrell, R., Cirignamo, L.,
Grazioso, R., Shah, K. S., Visser, G., Clajus, M.,
T¨umer, T. O., and Cherry, S. R. (2000). Design
studies of a high resolution PET detector using APD
arrays. IEEE Trans. Nuc. Sci., 47(3):1051–1057.
Thompson, C. J. , Murthy, K., Picard, Y. , Weinberg, I. N.
, Mako F. M. (1995) Positron Emission
Mammography (PEM): a promising technique to
detect breast cancer. IEEE Trans. Nucl. Sci. 42 1012–
1017.
BIODEVICES 2009 - International Conference on Biomedical Electronics and Devices
38