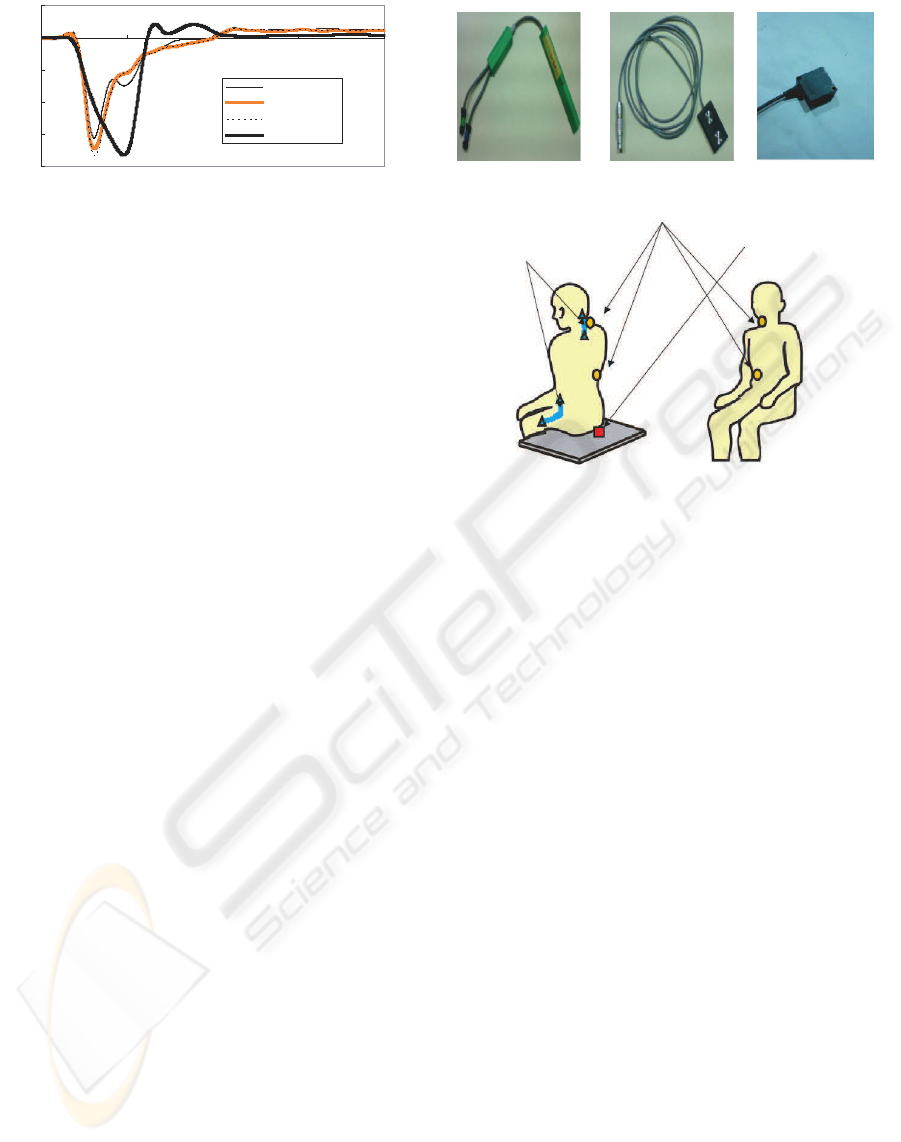
㪄㪏
㪄㪍
㪄㪋
㪄㪉
㪇
㪉
㪇 㪌㪇㪇 㪈㪇㪇㪇 㪈㪌㪇㪇 㪉㪇㪇㪇
㪚㪸㫉㫉㫀㪸㪾㪼㩷㪻㪼㫍㫀㪺㪼㪈
㪚㪸㫉㫉㫀㪸㪾㪼㩷㪻㪼㫍㫀㪺㪼㪉
㪚㪸㫉㫉㫀㪸㪾㪼㩷㪻㪼㫍㫀㪺㪼㪊
㪚㪸㫉
Figure 5: Comparison of seating surface acceleration of a
car and a carriage device.
The sudden stop experiment was carried out and the
seating surface acceleration was measured by an ac-
celerometer. A tensimeter was attached to the seatbelt
to measure the tension of the seatbelt. The trunk an-
gle, the cervical angle were measured by a goniome-
ter. The myoelectric potential of the sternocleidomas-
toid, the splenius, the abdominal and the muscle of
the back were measured by a myoelectric potential
sensor. Myoelectric potential sensors were attached
to the sternocleidomastoid, the splenius, the abdomi-
nal and the back muscles. To measure the sternoclei-
domastoid muscle, a myoelectric sensor was attached
between the sternoclavicularis and mastoid. To mea-
sure the splenius muscle, a myoelectric sensor was
attached one centimeter away from the fifth cervical
vertebra. To measure the abdominal muscle, a my-
oelectric sensor was attached three centimeters away
from the navel. To measure the back muscle, a my-
oelectric sensor was attached three centimeters away
from the fifth lumbar vertebra. Fig.6 shows the loca-
tion to attach the myoelectric sensors and goniome-
ters.
4 SIMULATION
4.1 Method
The muscle and physical parameters are need to be
determined in the muscle reflection model. The first
crash experiment was performed at a low acceleration
to obtain the human muscle parameters. Both the ex-
periment and the simulation results of the myoelectric
potential were matched to determine the human mus-
cle parameters(k, b,c, a
0
in Eq(7)). In addition to de-
termining the muscle parameter, another crash experi-
ment was performed at a high acceleration in order to
evaluate muscle reflection model. The human phys-
ical parameters(height, weight) were measured. The
trunk and cervical angle were simulated by Runge-
Kutta method which based on parameters obtained.
Input was the seating surface acceleration (A
x0
) and
(a)
(b)
(c)
(c)Accelerometer(b)Myoelectricsensor(a)Goniometer
(a)
(b)
(c)
(c)Accelerometer(b)Myoelectricsensor(a)Goniometer
Figure 6: Location to attach the myoelectric sensors, ac-
celerometers and goniometers.
outputs were the trunk and cervical angle and the my-
oelectric potential of measuring part.
4.2 Result
The relationship between simulation and experimen-
tal results of the trunk angle without/with seatbelt
is shown in Fig.7 and Fig.8. The relationship be-
tween simulation and experimental results of the my-
oelectric potential of the back without/with seatbelt is
shown in Fig.9 and Fig.10. Fig.7(a), (b), (c) and (d)
show that the trunk angle increases around 400ms and
returns to 0 degree. And, Fig.8(a’), (b’), (c’) and (d’)
show that trunk angle doesn’t incline. Therefore, the
simulation results of trunk angle without/with seat-
belt are similar to the experiment results in every sub-
ject. Fig.9(a), (b), (c) and (d) and Fig.10(a’), (b’),
(c’) and (d’) show that the simulation and experimen-
tal results of myoelectric potential increase around
400ms and return to 0 degree. And the peak time
of both results of myoelectric potential is matched.
Therefore, the motion and the muscle reflection of
disabled subjects could be simulated by the muscle
reflection model without/with seatbelt. And Fig.9(c),
(d) and Fig.10(c’), (d’) show that the muscle reflec-
tion of right/left hemiplegia subjects without seatbelt
is larger than that of using seatbelt.
COMPUTATIONAL MUSCLE REFLEX MODEL OF WHEELCHAIR USERS TRAVELING IN MOTOR VEHICLES -
Evaluation of the Motion and the Myoelectric Potential of People with Disabilities
83