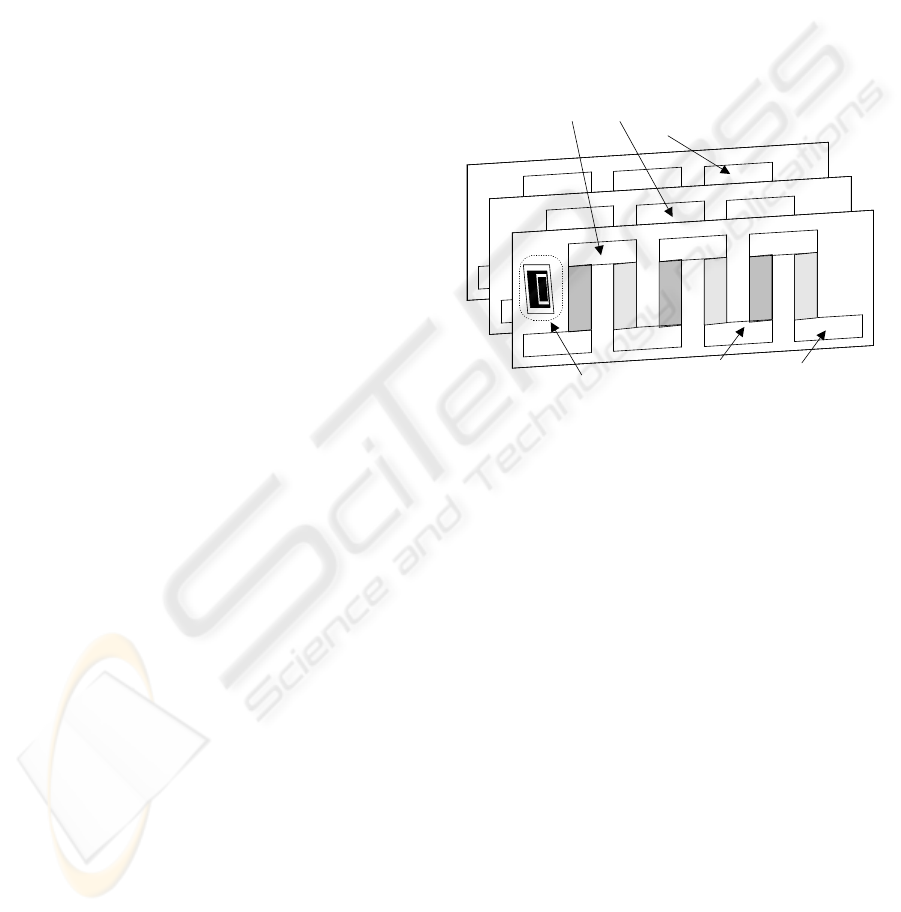
rechargeable thin-film battery of the Li-ion type
(integrated in the system). Ultra-low power
electronics performs DC-DC rectification with a
variable conversion factor and recharge the battery
on optimal conditions. Since a small volume is
required, integration into an IC is desirable. A
single-chip regulated thermoelectric power source is
the final goal to be achieved.
2 THERMOELECTRIC
GENERATOR
Future investigations, must prove if the operation
from low temperature gradients (a minimum
temperature difference of 3 ºC between ambient and
target thermo-source must provide an IC-compatible
voltage) and the power of 2 mW/cm
2
are possible to
be obtained. The fabrication of macro-scale
thermoelectric devices is based on standard
technologies for decades. Bismuth and antimony
tellurides was be used as thermoelectric materials
since these materials have the highest performance
figure-of-merit (ZT) at room temperature
(Gonçalves et al, 2006). The co-deposition method
was used to fabricate these thermoelectric thin films.
A very stable evaporation rate of each element
(Bi/Te and Sb/Te for the bismuth telluride and
antimony telluride, respectively) allows the
deposition of polycrystalline n-type and p-type
materials, when the substrate is heated in the range
200-300 ºC. The design of a thermoelectric
microdevice, with vertical microcolumns, connected
in series by metal contact areas, requires the
application of microsystem technologies
(Gonçalves et al, 2007). Reactive ion etching, lift-of
and wet-etching (hydrochloridric acid / nitric acid
solution) techniques were tested to create the vertical
columns. A thin (50 nm) layer of nickel (deposited
by e-beam) interfaces between the thermoelectric
material and the metal contact areas (1 µm of
aluminum), preventing the diffusion of the metal
from the contacts into the thermoelectric film. The
contact resistance plays a major role in the
performance of the device, and a value smaller then
1×10
-6
ohm.cm
2
was achieved. A silicon substrate
was used for integration with microelectronics,
while at same time providing good thermal contact
with heat source and sink. The fabricated battery, as
well as, all the electronic circuitry to receive the
energy and to recharge the thin-film integrated
Li-ion battery (open circuit voltages between 1.5 V
and 4.2V, maximum current of few mA/cm
2
with a
charge-storage capacity around 100 µAh/cm
2
), was
placed on the bottom side of the generator. Thin-film
solid-state batteries show a very high life cycle and
are intrinsically safe (Bates at al, 2000).
The Figure 1 shows an artist impression of a
thermoelectric microdevice, with vertical
microcolumns, connected in series by metal contact
areas. On this thermoelectric generator will be
placed the thin-film integrated battery and all the
electronic circuitry to receive the energy and to
recharge the battery. The application of this
thermoelectric scavenging energy systems, is in the
powering of a wireless EEG acquisition system.
Hot side junctions
Microbattery
Cold side junctions
Figure 1: An artist impression of a thermoelectric
microdevice.
3 EEG ACQUISITION SYSTEM
The standard wireless EEG solutions use a braincap
with wires running from the electrodes position to a
bulky central unity (amplification, signal filtering
and analog-to-digital conversion) (IMEC 2003). A
more interesting solution is to use compact wireless
EEG modules, where the electronics, the antenna
and each electrode are mounted together. The power
supply for these modules is obtained locally from
the thermoelectric generator. Also, it is possible to
integrate additional electronics (amplification,
filtering and high-resolution digital conversion), for
local signal processing in these small-size individual
wireless EEG modules.
Bipolar or unipolar electrodes can be used in the
EEG measurement. In the first method the potential
difference between a pair of electrodes is measured.
In the second method, the potential of each electrode
is compared, either to a neutral electrode or to the
average of all electrodes. Figure 2 shows the full
block diagram of the wireless EEG module, where it
A WIRELESS EEG ACQUISITION SYSTEM WITH THERMOELECTRIC SCAVENGING MICRODEVICE
381