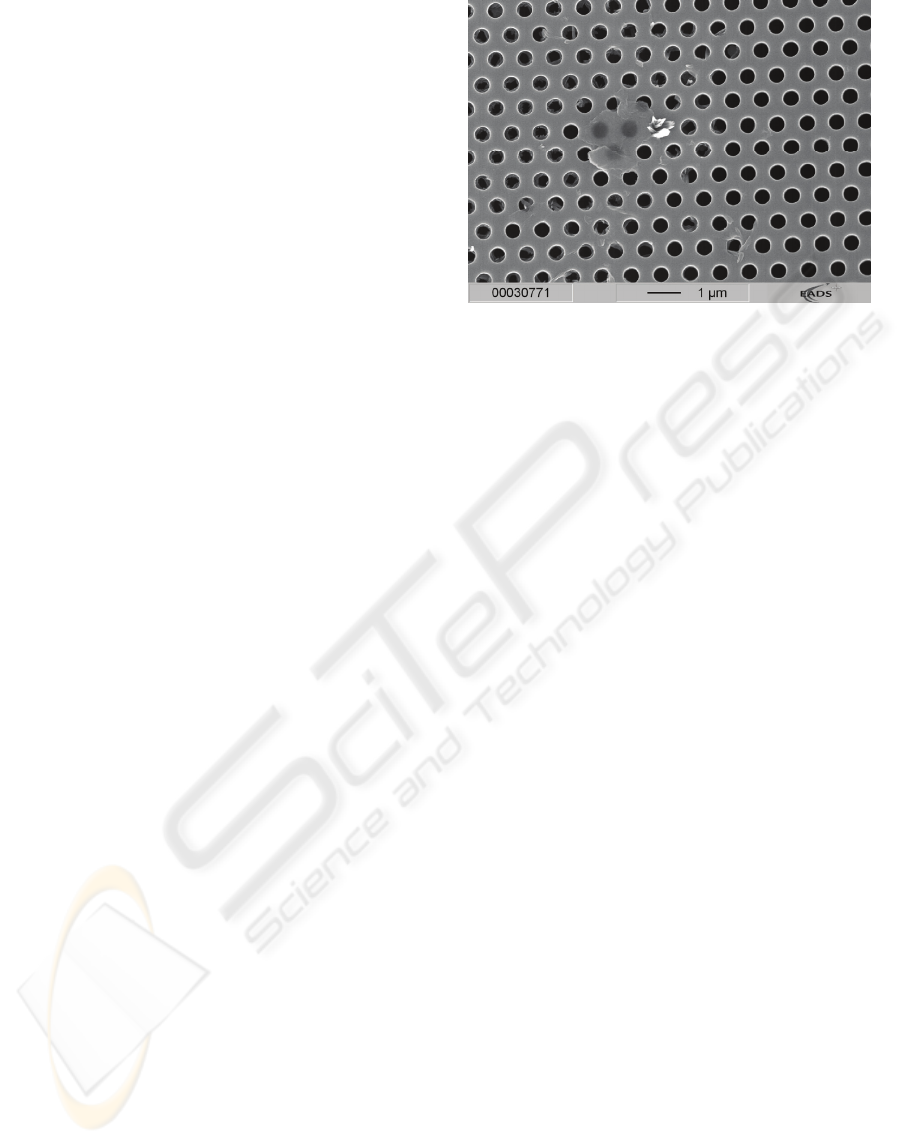
be effectively captured and retained on the filter
surface. Once on the filter, they are easily accessible
to any kind of specific or non-specific staining
method (e.g. with DNA intercalating dyes, enzyme
substrates, membrane stains, labelled antibodies or
hybridization probes). Fluorescent labels are used in
our monitoring equipment.
Micromechanical filters are highly discriminative
and show a much better filtration performance
(higher filtration rate) than conventional filters of the
same size. Their absolutely flat surface does not
allow any particle to penetrate deeper into the filter’s
structure. Any bacteria retained by the filter will be
present at the surface, allowing easy detection and
washing off afterwards. Some work has been
reported on using microfilters which successfully
separate particles by means of filtration (Hsiai,
2002; Xing, 1999). These filters have pore diameters
of 6-12 µm. Ogura et al. reported the separation of
deformed red blood cells using microfilters with
larger pore size (Ogura, 1991a; b). Since the average
size of bacteria, for example E. coli, is
approximately 1µm, theses filters are not suitable for
the discriminative enrichment of bacteria.
In this paper we describe the successful capture,
labelling and detection of E. coli on the surface of
micromechanical sieves. We have integrated the
MEMS filters into a microfluidic system, allowing
fully automated water analysis with this biosensor
system.
2 MATERIALS AND METHODS
2.1 Capture and Enrichment of
Bacteria
The micromechanical filters are composed of a
Si
3
N
4
membrane (5 x 5 mm, approx. 1 µm thick)
supported by a silicon frame. The membrane is
perforated, each pore with a diameter of 450 nm
(Figure 1). In contrast to tissue or membrane based
filters, particles are not trapped inside a three
dimensional filter structure but on the surface.
Therefore, the particles are directly accessible.
Previous experiments show that bacteria trapped on
the surface can be easily removed after filtration
(Reidt, in press). A further advantage of the
micromechanical filters is based on the fact that all
pores are simultaneously etched after a
photolithographic patterning step. This ensures that
all pores have exactly the same diameter and their
spacing is completely homogenous (Figure 1).
Figure 1: REM picture of a micromechanical filter surface
with 0,45 µm diameter pore size (top view) after filtration
of drinking water.
2.2 A Fully Automated System for
Water Analysis
Using the micromechanical filters (sieves), we have
developed a fully automated system for capturing,
labelling and detecting bacteria in water. The sieves
are housed in a microfluidic chamber with a glass
window in very close distance above the filter
surface. There is a water inlet close to the filter and
one outlet in opposite position. Filter and glass
window form a microfluidic channel, where liquid
can be pumped from the inlet to the outlet passing in
parallel to the filter surface (cross flow). A second
outlet is positioned on the back side of the filter,
allowing for flow through (dead end) filtration.
Using a double selector valve, inlet and outlets can
be activated to perform both types of flow. There is
also the possibility to swap inlet and outlet for back
flushing the filter (Figure 2). The maximum
differential pressure over the microsieve is limited to
2 bar to avoid breaking the filter.
For detection, we use a standard setup with
coaxial excitation and emission light paths. Light
from a high power LED is collected and passed
through an emission filter (485 +/-12 nm band pass).
It is then reflected at 45° angle by a dichroic mirror
(505 nm) and focussed onto the filter surface. The
fluorescent light passes the mirror, is filtered
through a 520 +/-15 nm band pass filter and
focussed onto a photomultiplier tube (Figure 3).
Device control and data acquisition is performed by
LabView software on a laptop computer.
The precision pump (0 - 10 bar, 0.01 - 70
mL/min) was purchased from HNP (Parchim,
Germany), whereas the selector valves were
obtained from Valco-VICI (Schenkon, Switzerland).
As detector we use a Perkin-Elmer MP962 photo
A NOVEL MOBILE MONITORING SYSTEM FOR FAST AND AUTOMATED BACTERIA DETECTION IN WATER
385