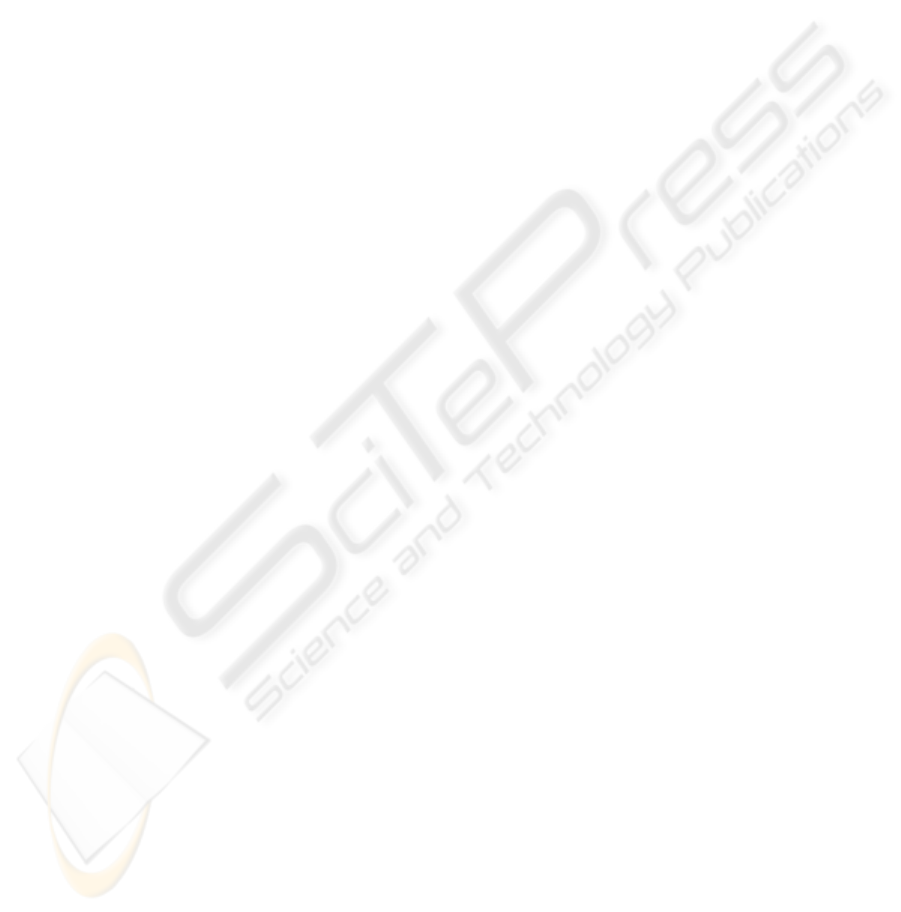
participants. However, the ICC should be interpreted
with caution. Bland and Altman 95% limits of
agreement are independent of the true variability in
the observations and therefore, complement the ICC
analysis and provide detail regarding the nature of
the observed intra-subject variability (Rankin and
Stokes, 1998). The reliability assessed from Bland
and Altman techniques was found to be acceptable,
and no consistent bias was detected in any recording
position of the two crackles’ variables studied.
Therefore, crackle characterisation using CALSA
was deemed reproducible over short time periods.
The ICC has rarely been used when analysis lung
sounds, but ‘satisfactory’ reproducibility of lung
sounds based on ICC results have been reported
(Schreur et al., 1994). However, this analysis was
performed in the frequency domain where spectral
characteristics were considered and the recordings
were performed in a sound proofed room.
In this research, the SRD values, over short time
periods, for both variables studied (crackles’ IDW
and 2CD) presented a similar range of values
indicating the stability of the measure in CF
participants. Smallest real difference calculations
were not found in other published studies involving
lung sound analysis and therefore, comparisons with
the findings of this research were not possible.
As it has been demonstrated, the use of CALSA
in terms of clinical properties shows potential to be
use as an outcome measure for respiratory therapy
but this has not been previously explored. At this
point in time, the data related to lung sounds are
complex and time consuming to analyse. To be
clinically useful as an outcome measure it will be
essential to simplify, and increase the speed of, the
analytical process. Furthermore the cost-
effectiveness of implementing this outcome measure
is unknown. Validation of CALSA as a responsive
outcome measure is challenging because of the lack
of a gold standard respiratory therapy measure with
which to compare it. Studies relating to
responsiveness to change of lung sounds before and
after an intervention of known effect e.g.
bronchodilators, suction, would help in clarifying
the responsiveness of the measure, and increasing
understanding of the validity of CALSA in clinical
settings. Nevertheless, the aims proposed at the
outset for this research have been achieved.
5 CONCLUSIONS
This preliminary study suggests that the use of
CALSA to characterise crackles is reproducible over
short time periods in cystic fibrosis outpatients. This
finding gives initial confidence that CALSA has
potential as an outcome measure for respiratory
therapy interventions (such as physiotherapy for
airway clearance) but further evaluation is
necessary.
ACKNOWLEDGEMENTS
We would like to thank the Fundação para a Ciência
e Tecnologia (FCT), Portugal, for funding one of the
authors (Alda Marques) during her PhD studies.
REFERENCES
A.T.S. (1977) Update nomenclature for membership
relation. ATS News, 3, 5-6.
Baughman, R. P. & Loudon, R. G. (1984) Quantitation of
wheezing in acute asthma. Chest, 86, 718-722.
Bland, J. M. & Altman, D. G. (1986) Statistical methods
for assessing agreement between two methods of
clinical measurement. Lancet, 1, 307-310.
Brooks, D., Wilson, L. & Kelsey, C. (1993) Accuracy and
reliability therapists in auscultating tape-recorded lung
sounds. Physiother Canada, 45, 21-24.
Chen, S.-C., Chang, K.-J. & Hsu, C.-Y. (1998) Accuracy
of auscultation in the detection of
haemopneumothorax. European Journal Surgery, 164,
643-645.
CSP (2002) Priorities for Physiotherapy Research in the
UK: Topics prioritised by the cardiorespiratory expert
panel (Annex 1), London, Chartered Society of
Physiotherapy.
Earis, J. E. & Cheetham, B. M. G. (2000) Current methods
used for computerized respiratory sound analysis.
European Respiratory Review, 10, 586-590.
Elphick, H. E., Lancaster, G. A., Solis, A., Majumdar, A.,
Gupta, R. & Smyth, R. L. (2004) Validity and
reliability of acoustic analysis of respiratory sounds in
infants. Archives Disease Childhood, 89, 1059-1063.
Fleiss, J. (1986) Reliability of measurements, New York,
John Wiley & Sons.
Forgacs, P. (1978) Lung sounds, London, Bailliere
Tindall.
Fredberg, J. & Holford, S. K. (1983) Discrite lung sounds:
crackles (rales) as stress-relaxation quadrupoles.
Journal of Acoustic Society of America, 73, 1036-
1046.
Gavriely, N., Nissan, M., Cugell, D. W. & Rubin, A. H. E.
(1994) Respiratory health screening using pulmonary
function tests and lung sound analysis. European
Respiratory Journal, 7, 35-42.
Kiyokawa, H., Geenberg, M., Shirota, K. & Pasterkamp,
H. (2001) Auditory detection of simulated crackles in
breath sounds. Chest, 119, 1886-1892.
COMPUTER AIDED LUNG SOUND ANALYSIS - A Preliminary Study to Assess its Potential as a New Outcome
Measure for Respiratory Therapy
255