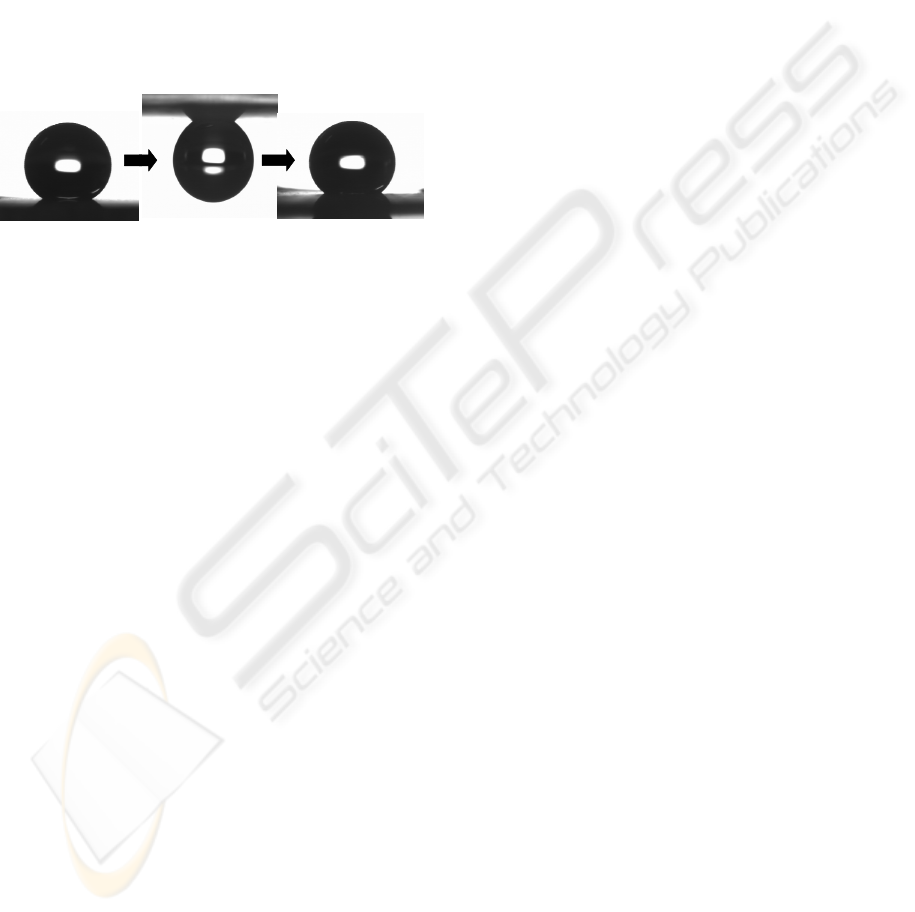
density of 15% possessed a WCA of 150° and a SA
of 30° (a middle-adhesion MP surface). The MP
surface with dome density of 25% possessed a WCA
of 145° and a not measured SA because the droplet
adhered the surface when turned upside down (a
high-adhesion MP surface). As the dome density
was increasing, the WCA was decreasing and the SA
was increasing, which means that the hydrophilic
nickel domes gave the adhesion behaviors. This
result made clear that the adhesion property was
controlled by the quantity of the nickel dome easily
changed by the catalytic mixture solution
temperature for electroless plating.
155° 150°
Low-adhesion surface
HIgh-adhesion surface
Middle-adhesion surface
155° 150°
Low-adhesion surface
HIgh-adhesion surface
Middle-adhesion surface
Figure 5: Droplet transfer of 5.0-mg water droplet from
the low-adhesion MP surface to the middle-adhesion MP
surface via the high-adhesion MP surface.
Water micro droplet transfer was attempted by
using the MP surfaces with different adhesion
properties as shown in Figure 5. A water droplet of 5
mg on the low-adhesion MP surface was carried
with the high-adhesion MP surface by means of
pulling off after slight contact from above. However,
in the case of the middle-adhesion MP surface, a
water droplet did not remove from the low-adhesion
MP surface. On the other hand, the high-adhesion
MP surface was not caught a water droplet on the
middle-adhesion MP surface from above. These
results suggest that the adhesion force of the high-
adhesion MP surface was stronger than that of the
low-adhesion MP surface plus gravity on the water
droplet, and weaker than that of the middle-adhesion
MP surface plus gravity on the water droplet. By
using this difference, the water droplet was
transferred from the low-adhesion MP surface to the
middle-adhesion MP surface via the high-adhesion
MP surface. After transfer, the water droplet on the
middle-adhesion MP surface was sliding when the
surface was tilted at about 30°. A droplet transfer
reported in the past is from a superhydrophobic
surface to a hydrophilic surface via an adhesion
superhydrophobic surface (Cho, 2008). Therefore,
after transfer, the water droplet is spreading, and is
unable to be handled. The novel transfer method in
this report remains a droplet shape after transfer, so
that the droplet was handily manipulated again.
These behaviors were useful to microfluidic devices,
bio interfaces, and micro-reactors.
4 CONCLUSIONS
We could fabricate water repellency and adhesion
properties of superhydrophobic metal-polymer
surfaces by electroless plating for self-organized
honeycomb films including immersion in a catalytic
Pd salt and a cationic polymer mixture solution. It
was found that a water contact angle and a water
droplet adhesion property were changed by metal
dome density which was easily controlled by the
temperature of the catalytic mixture solution.
Droplet transfer between superhydrophobic surfaces
was demonstrated by means of using the metal-
polymer surfaces with different adhesion properties.
REFERENCES
Cho, W. K., & Choi, I. S. (2008). Fabrication of hairy
polymeric films inspired by geckos: wetting and high
adhesion properties. Advanced Functional Materials,
18, 1089−1096.
Feng, L., Zhang, Y., Xi, J., Zhu, Y., Wang, N., Xia, F., &
Jiang, L. (2008). Petal effect: a superhydrophobic state
with high adhesive force. Langmuir, 24, 4114−4119.
Hosono, E.,Fujihara, S., Honma, I., & Zhou, H. (2005).
Superhydrophobic perpendicular nanopin film by the
bottom-up process. Journal of the American Chemical
Society, 127, 13458−13459.
Ishii, D., Yabu, H., Shimomura, M. (2008). Selective
metal deposition in hydrophobic porous cavities of
self-organized honeycomb-patterned polymer films by
all-wet electroless plating. Colloid and Surface; A
313–314, 590–594.
Ishii, D., Udatsu, M., Iyoda, T., Nagashima, T., Yamada
M., & Nakagawa, M. (2006). Electroless deposition
mechanisms on fibrous hydrogen-bonded molecular
aggregate to fabricate Ni-P hollow microfibers.
Chemistry of Materials. 18, 21522158.
Karthaus, O., Maruyama, N., Cieren, X., Shimomura, M.,
Hasegawa, H., & Hashimoto, T. (2000). Water-
assisted formation of micrometer-size honeycomb
patterns of polymers. Langmuir, 16, 6071−6076.
Onda, T., Shibuichi, S., Satoh, N., & Tsujii, K. (1996).
Super-water-repellent fractal surface. Langmuir, 12,
2125−2127.
Yabu, H., Takebayashi, M., Tanaka, M., & Shimomura,
M. (2005). Superhydrophobic and Lipophobic
Properties of Self-Organized Honeycomb and
Pincushion Structures. Langmuir, 21, 3235−3237.
Zhang, X., Shi, F., Niu, J., Jiang, Y., & Wang, Z. (2008).
Superhydrophobic surfaces: from structural control to
functional application. Journal of Materials Chemistry,
18, 621−633.
BIODEVICES 2009 - International Conference on Biomedical Electronics and Devices
116