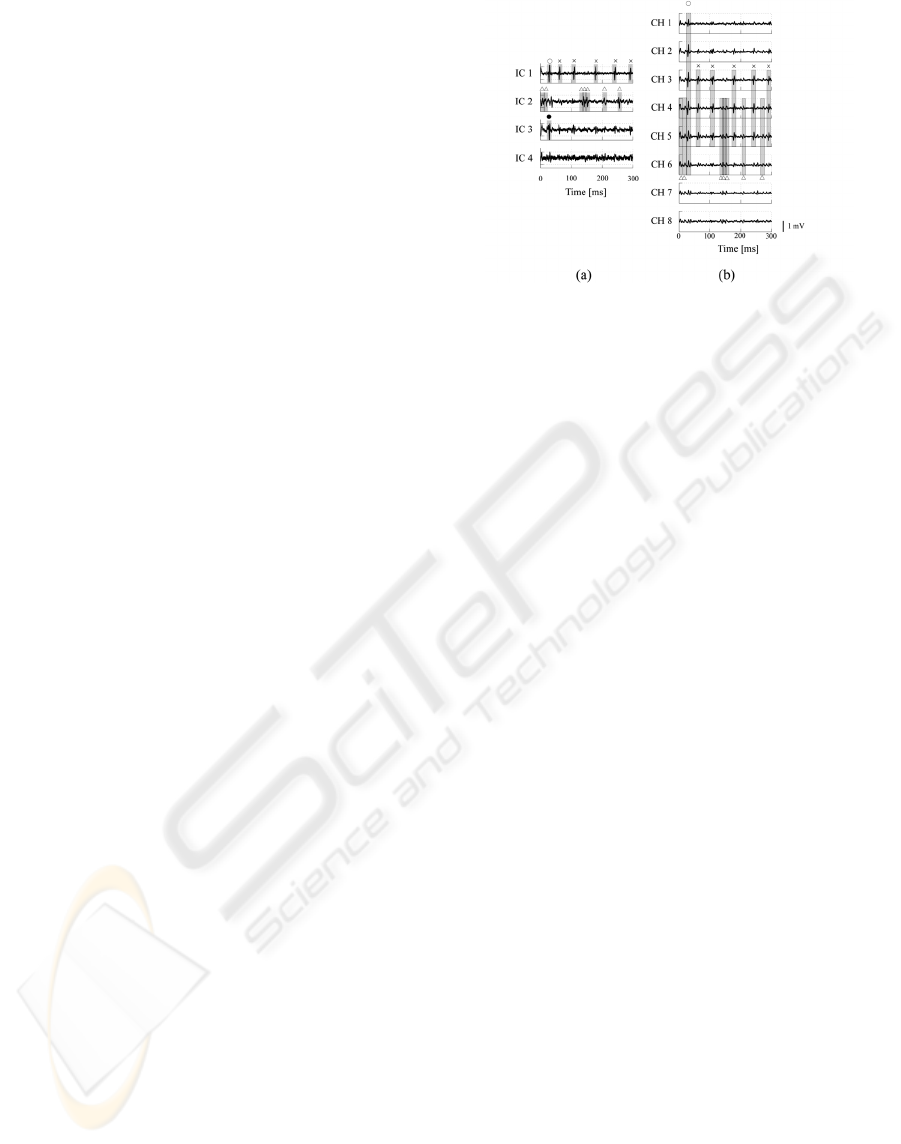
3.2 Result with Consideration of
Electrode Orientation
Apart from insufficient, we tried to obtain better
results with repeating measurement three times with
changing the electrode orientation. Isometric con-
traction was at 5% MVC and the duration 15 (s).
The result of ICA is shown in Fig. 5 (a) and the
corresponding measured SEMG in Fig. 5 (b). In IC
1, SMUAP-like signals marked with × could be
found clearly; briefly the corresponding MU is
called MU 2. The larger amplitude signal marked
with open circle ○ was also found in IC 1; the cor-
responding MU is called MU 1. In IC 3, a large
amplitude SMUAP-like signal marked by ● was
found. This MU might correspond to MU 1 because
of appearance at the same time in both IC 1 and IC 3.
Consequently the feature extraction of MU 2 was
effectively sufficient in IC 1, and small amplitude
signal corresponding to MU 3 was found in IC 2 as
shown with triangle △. Focusing attention on IC 1
and IC 3, MUs marked by ● and ○ are the same
MU 1. This conclusion could be supported by com-
paring the SEMG signal in Fig. 5 (b) with ICA in
Fig. 5 (a). SMUAPs of MU 1 are clearly seen from
CH 1 to CH 6 in Fig. (b) and those of MU 2 from
CH 3 to CH 5. It should be noted that both SMUAPs
of MU 1 and MU 2 in CH 5 are very similar in the
shape, which means that decomposition of SMUAP
is difficult in judging from only the SEMG signal.
4 CONCLUSIONS
In this study, effects of electrode orientation on the
result of ICA was analyzed with simulation study
and actual voluntary isometric contraction of FDI.
Obtained results were as follows. When the long
axis of the eight-channel electrode was perpendicu-
lar to the long axis of muscle fiber, the result of ICA
was best in terms of the feature extraction of
SMUAP; large amplitude of SMUAP-like signals of
the single MUs appeared in one component of ICA.
As the orientation of the electrode changed apart
from this direction, unexpected results of ICA were
obtained; i.e., large amplitude of SMUAP-like sig-
nals of the single MUs appeared almost at the same
time in other components of ICA, and SMUAP-like
signals of different MUs appeared in one component.
Figure 5: Result of ICA (a) and measured SEMG (b) at
5% MVC of isometric contraction.
REFERENCES
Xu, Z., Xiao, S., and Chi, Z.: ART2 neural network for
surface EMG decomposition, Neural Computing &
Applications, vol. 10(1), (2001) 29-38.
Bonato, P., Erim, Z., and Gonzalez-Cueto, J.: Decomposi-
tion of superimposed waveforms using the cross time
frequency transform, Proc. 23rd Ann. Int. Conf. IEEE
EMBS, Istanbul, (2001).
Hyvarinen, A., Karhunen, J., and Oja, E.: Independent
component analysis, Hoboken: John Wiley and Sons,
The publishing company. London, 2
nd
edition, (2001).
Maekawa, S., Arimoto, T., Kotani, M., and Fujiwara, Y.:
Motor unit decomposition of surface EMG using mul-
tichannel blind deconvolution, Proc. XIVth Congress
of ISEK, Vienna, (2002) 38-39.
Nakamura, H., Yoshida, M., Kotani, M., Akazawa, K., and
Moritani, T.: The application of independent compo-
nent analysis to the multi-channel surface electromyo-
graphic signals for separation of motor unit action po-
tential trains: part I-measuring techniques, J Electro-
myogr Kinesiol., vol. 14, (2004) 423-432.
Gonzalo, A., Okuno, R., and Akazawa, K.: A decomposi-
tion algorithm for surface electrode-array electromyo-
grams, IEEE Engineering in Medicine and Biology
Magazine, vol. 24(4), (2005) 63-72.
Griep, P., Gielen, F., Boom, H., Boon, K., Hoogstraten, L.,
Pool, C., and Wallinga-De-Jonge, W.: Calculation and
registration of the same motor unit action potential”,
EEG and Clinical Neurophysiol., vol. 53, (1982) 388-
404.
Disselhorst-klug, C., Silny, J., and Rau, G.: Estimation of
the relationship between the noninvasively detected
activity of single motor units and their characteristic
pathological changes by modeling, J Electromyogr
Kinesiol., vol. 8, (1998) 323-335.
Akazawa, J., Sato, T., Minato, K., and Yoshida, M.: Me-
thod of estimating location and territory of motor units
in human first dorsal interosseous muscle with multi-
channel surface electromyograms, JSMBE, vol. 43(4),
(2005) 595-604. (in Japanese)
BIOSIGNALS 2009 - International Conference on Bio-inspired Systems and Signal Processing
424