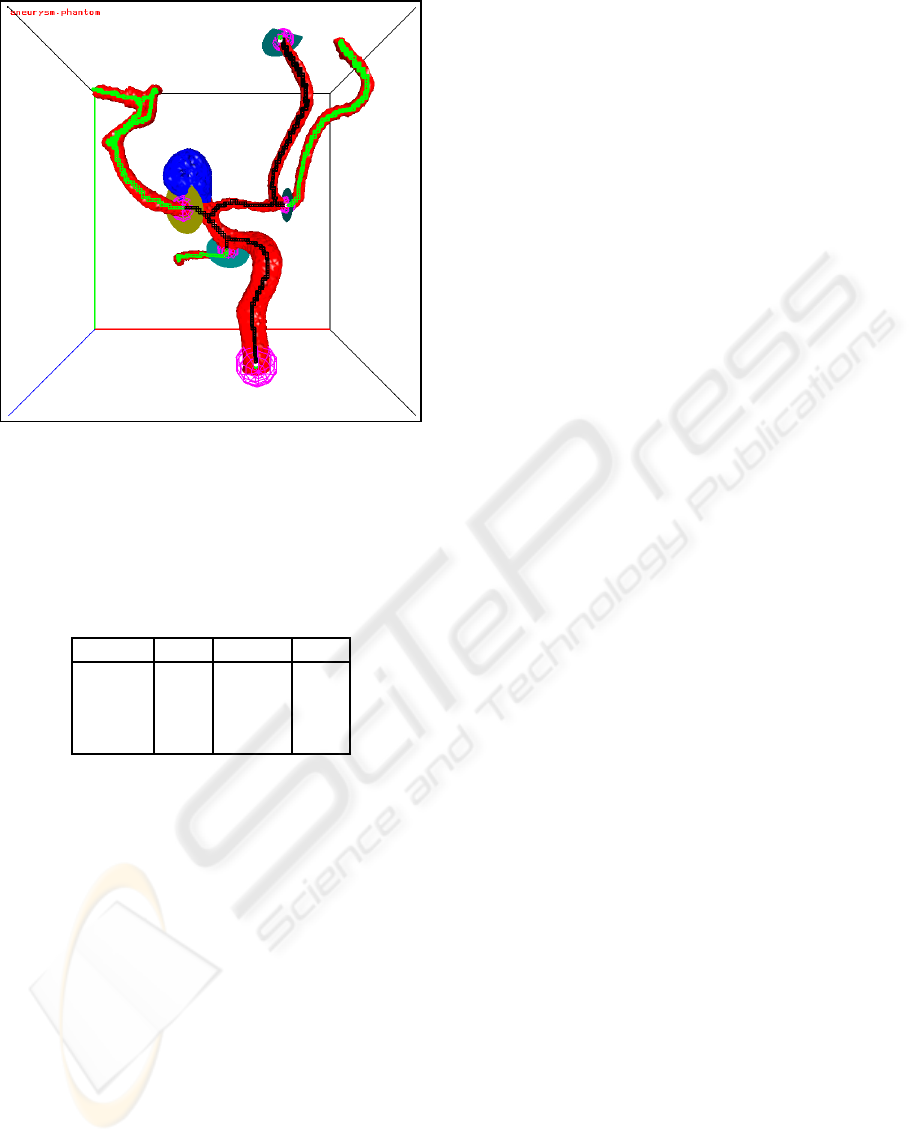
Figure 16: The closed flow section for probes 1 and 3.
The skeleton voxels of the flow section are black, the other
skeleton voxels are green.
Table 3: The characteristics of the number of extremity
probes NE, the number of tests NT and the resulting num-
ber of blocking probes NB including the two initial probes
for fully-automatic geometry closure of 11869 open flow
sections
NE NT NB
min. 3.0 3.0 3.0
median 15.5 112.5 14.0
mean 19.2 247.3 14.8
max. 56.0 1540.0 48.0
8 CONCLUSIONS
The following conclusions can be drawn from the re-
sults, the figures and the experiences gathered during
testing:
1. Fully-automatic geometry closure of an aneurysm
gives always correct (i.e. a single connected
closed flow section) and visually acceptable re-
sults.
2. Fully-automatic geometry closure of an open flow
section gives correct and visually acceptable re-
sults except when multiple closed flow sections
arise from the initial boundary probes.
3. Preparing patient specific geometries for compu-
tational fluid dynamics is a time-consuming and
error-prone task. The work above is the first to au-
tomatically create and validate an error free closed
simulation domain. It has been implemented in
a simulation and visualization software environ-
ment that allows a user to prepare a simulation in
a matter of minutes instead of an hour of work.
4. Whether flow sections, generated by fully-
automatic geometry closure of an aneurysm based
on a length criterion, are suitable for hemodynam-
ics simulations has yet to be investigated.
REFERENCES
Bruijns, J. (2001). Fully-automatic branch labelling of
voxel vessel structures. In Proc. VMV, pages 341–350,
Stuttgart, Germany.
Bruijns, J., Peters, F., Berretty, R., and Barenbrug, B.
(2007). Fully-automatic correction of the erroneous
border areas of an aneurysm. In Proc. BVM, pages
293–297, Muenchen, Germany.
Bruijns, J., Peters, F., Berretty, R., van Overveld, C., and ter
Haar Romeny, B. (2005). Computer-aided treatment
planning of an aneurysm: The connection tube and the
neck outline. In Proc. VMV, pages 265–272, Erlangen,
Germany.
Butty, V., Gudjonsson, K., P.Buchel, Makhijani, V., Ven-
tikosa, Y., and D.Poulikakos (2002). Residence times
and basins of attraction for a realistic right inter-
nal carotid artery with two aneurysms. Biorheology,
39:387–393.
Castro, M., Putman, C., and Cebral, J. (2005). Computa-
tional modeling of cerebral aneurysms in arterial net-
works reconstructed from multiple 3d rotational an-
giography images. In Proc. SPIE: Medical Imaging,
volume 5746, pages 233–244, San Diego, CA, USA.
Cebral, J., Castro, M., Millan, D., Frangi, A., and Putman,
C. (2005). Pilot clinical investigation of aneurysm
rupture using image-based computational fluid dy-
namics models. In Proc. SPIE: Medical Imaging, vol-
ume 5746, pages 245–256, San Diego, CA, USA.
Moret, J., Kemkers, R., de Beek, J. O., Koppe, R., Klotz,
E., and Grass, M. (1998). 3D rotational angiography:
Clinical value in endovascular treatment. Medica-
mundi, 42(3):8–14.
Philips-Medical-Systems-Nederland (2001). INTEGRIS
3D-RA. instructions for use. release 2.2. Techni-
cal Report 9896 001 32943, Philips Medical Systems
Nederland, Best, The Netherlands.
Venugopal, P., Duckwiler, G., Valentino, D., Chen, H.,
Villablance, P., Vinuela, F., Kemkers, R., and Haas,
H. (2005). Correlating aneurysm growth to hemody-
namic parameters: the case of a patient-specific ante-
rior communicating artery aneurysm. In Proc. SPIE:
Medical Imaging, volume 5746, pages 780–791, San
Diego, CA, USA.
Waechter, I., Bredno, J., Hermans, R., Weese, J., Barratt, D.,
and Hawkes, D. (2008). Evaluation of model-based
blood flow quantification from rotational angiography.
In Proc. SPIE: Medical Imaging, volume 6916, San
Diego, CA, USA.
VISAPP 2009 - International Conference on Computer Vision Theory and Applications
162