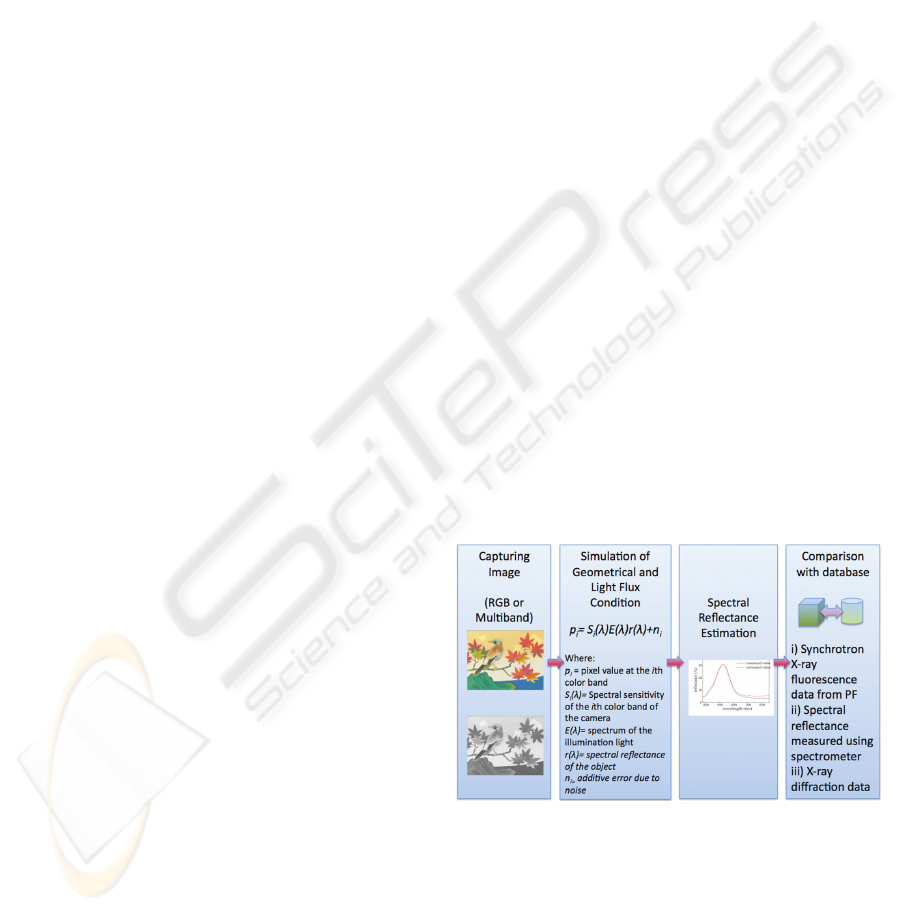
certain range (Balas, et al., 2003). In other
wavelength range, this is more pronounced.
However, at the VL-NI range the interaction is more
complex. Nonetheless, there are some unique
features that are only observable within this range.
This makes it valuable in cultural heritage analysis.
2 EXPERIMENT
2.1 Imaging and Spectroscopic
Synchrotron Radiation Analysis
Synchrotron radiation X-ray fluorescence (SRXRF)
was performed on a 13
th
century Mongolian textile
and dislodged fragments collected from an old
Korean painting. Measurements were done at beam
line 4A of Photon Factory. The electron beam
energy in the storage ring was 2.5 GeV, with a
maximum current of 400 mA. Incident X-ray
energy was 15 keV. The cross-section of the beam
was approximately 1(v) x 1(h) mm
2
on the sample.
The synchrotron radiation was monochromated by a
multilayered reflecting mirror. Precise beam size of
monochromated X-rays was adjusted using slits. The
incident and transmitted X-rays were monitored by
ionization chambers that were set in front of and
behind the sample. The fluorescent X-rays were
collected by a solid-state detector at 90 degrees to
the incident beam. Measurements were performed in
air. Point spectra were measured for obtaining
consistent elements of the samples. The spectra were
obtained by using a multi-channel analyzer. The
measurement time was 100 seconds for each
spectrum. XRF imaging technique was applied in
order to investigate the distributions of main
elements. X-Y step pulse motors moved the sample
stage. The measurement areas were divided into
matrices of 20 x 20 pixels. At each pixel, the XRF
yields for each element were integrated by single
channel analyzers. The measurement time was three
seconds for each pixel.
Synchrotron radiation was also used to
investigate the relationship between fine structural
change and color fading in natural mineral,
specifically azurite and malachite. Heating of natural
pigments is well practiced among traditional
Japanese painters to modify the shade and color of
the pigments. It is of great interest to understand the
factors involved in this process. Ten samples of each
pigment were heated at 260°C with holding time
from 10 minutes up to 90 minutes at 10-minute
increments. The spectral reflectance of the heated
and unheated pigments was measured to track the
changes in color. X-ray fluorescence and X-ray
absorption fine structure (XAFS) were used to
characterize the pigments. The incident X-ray was
15 keV for XRF analysis while the energy was
scanned at the Cu K absorption edge from 8.90 to
9.09 keV for XAFS.
2.2 Imaging at the Visible-Near
Infrared Range
In order to use VL-NIS for the analytical imaging of
cultural heritage, a technique for image analysis was
developed. Figure 1 illustrates the basic scheme of
the analysis. It begins by capturing an image of the
object to capture spectral and color information. The
picture can be an RGB or a multispectral image. An
RGB image refers to an image captured using
tristimulus values corresponding to red, green and
blue colors. A multispectral image, on the other
hand, is an image captured by using 6-7 bands of
color and infrared filters. The images are captured
within a certain wavelength band. The color
information is then used to simulate the geometrical
and light flux conditions. The simulation enables the
estimation of the spectral reflectance, which is used
for comparison with a database to provide useful
information about the image. The database includes
more than 1000 mineral pigments and is
continuously being updated. The database also
includes additional data such as SRXRF spectra,
XRD spectra as well as information about the
artwork’s history.
Figure 1: Image analysis scheme using VL-NIS.
IMAGAPP 2009 - International Conference on Imaging Theory and Applications
122