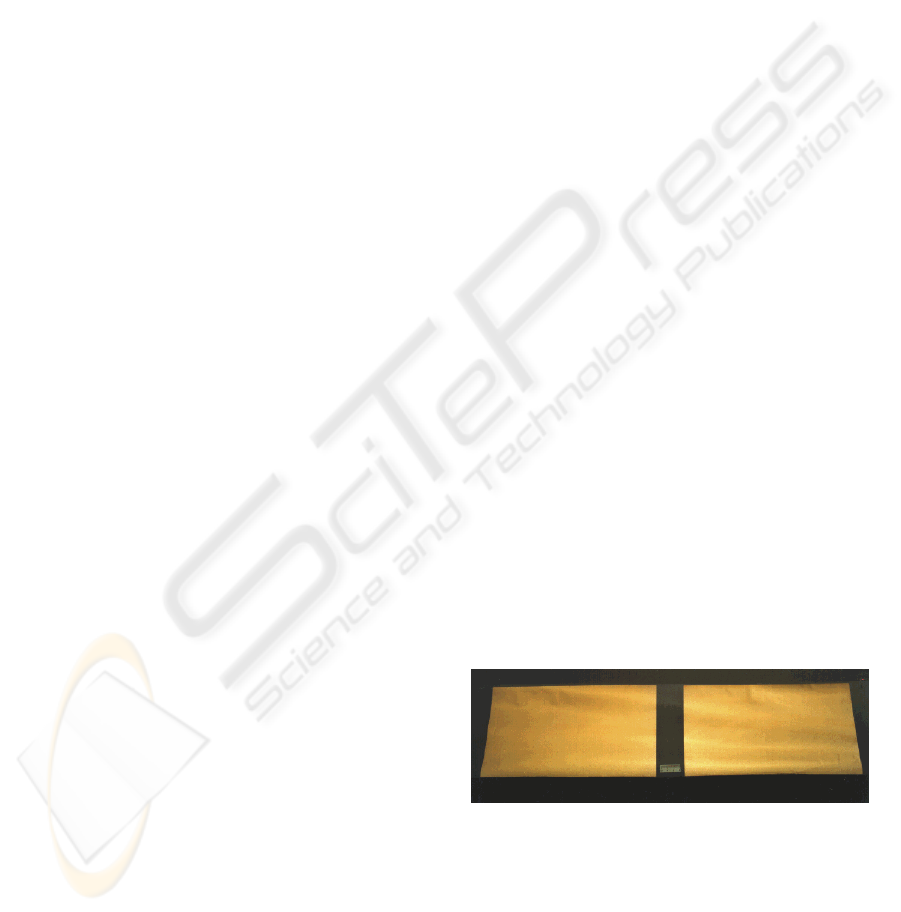
Infrared Thermography Cameras. Infrared
Thermography is a technique for carrying out
inspections and non-destructive tests which has
multiple applications in the development of
machines and products, equipment and facilities
maintenance, and troubleshooting.
Since all bodies emit (according to their
temperature) infrared radiation, which increases in
intensity as the temperature rises, variations in this
intensity can be detected by using infrared sensors.
Thermal cameras can detect radiation in the
infrared range of the electromagnetic spectrum
(usually between a 900 and 14000 nm wavelength,
instead of operating in the visible range of 450 to
750 nm) and can produce images of this radiation.
These cameras are fitted with a sensor matrix
(called microbolometer) that can be developed using
pyroelectric materials. Depending on the intensity of
the radiation more or less current is sent to the
camera’s control electronics, which with the aid of
specific software enables temperature maps to be
obtained.
Some of the fundamental advantages of the
technique are its speed and ease of use, easy to
interpret temperature map-based results and the fact
that it is a non-destructive technique that does not
damage the systems under study (Schindel, 2007,
Maldague, 2001).
Apart from these applications, its use as a
support tool for developing medical devices,
especially those based on the use of thermal
materials has also been proposed (Paumier, 2007).
Biometric Systems. Pyroelectric materials can also
be used as part of complex biometric systems for
real-time recognition of people inside a building or
room with security purposes, or in the medical field
for evaluating the progress of injuries that limit the
mobility of patients (Fang, 2007).
Aided Surgery. These materials have also been
proposed and tested for measuring blood
temperature during surgeries, such as coronary stent
placements, with the purpose of relating temperature
profile with the blood velocity field and using this
comparison as a method of controlling the surgical
procedure (Mochi, 2004).
Flow Sensors. Dymedix Co. has developed nasal
flow sensors using PVDF, that besides being
piezoelectric has also pyroelectric properties and can
be used as temperature sensor. This products allow
an active management of pathologies such as sleep
apnea or sudden death in children. Such devices are
placed adjacent to the nostrils and patient breath
induces charges to the sensor, with a typical and
recognisable pattern. When breathing ceases, the
pattern changes and the microcontroller detects such
problem and activates an alarm to alert both the
patient and his or her relatives.
X-Ray Intensity Sensors. Based on corporal
heating due to absorption of X-Ray (during
radiological explorations) pyroelectric sensors can
be used, so as to make an estimation of the dosage
received and in order to avoid risk situations. The
phenomenon has been proved “in vivo” during
mammography scans with positive results according
to precision and sensitivity (De Paula, 2005).
3 PVDF PYROELECTRIC
POLYMER SENSORS
Polyvinylidene fluoride or PVDF -(CH
2
-CF
2
)-
n
and
its co-polymers such as poly(vinilydenefluoride-
trifluoroethylene) or P(VDF-TrFE), are the polymers
of this kind with the largest number of industrial
applications. They posses partial crystalinity with an
inactive amorphous phase and an elastic modulus
close to between 1 and 10 GPa.
The ferroelectric structure makes this polymer both
piezoelectric and pyroelectric, which increases its
applications, not only as temperature and pressure
sensor, but also as actuator. Its use as actuators is
limited by the need to apply high electric fields
(around 20 V/μm for a 3% deformation), but their
use as pressure sensors is taking the place of
traditionally used piezoelectric ceramic materials.
Regarding pyroelectricity its important value of
pyroelectric coefficient, together with its greater
resistance and sensitivity is displacing the use of
pyroelectric ceramics.
Figure 1: Metallized PVDF sheets. Piezotech S.A..
To make the sensors, we took PVDF 40 μm
thick sheets from Piezotech S.A. with Au-Pt coated
electrodes. These sheets were cut, joined to the
connecting wires and suitably encapsulated into
flexible polyurethane layers to protect them.
BIODEVICES 2009 - International Conference on Biomedical Electronics and Devices
454