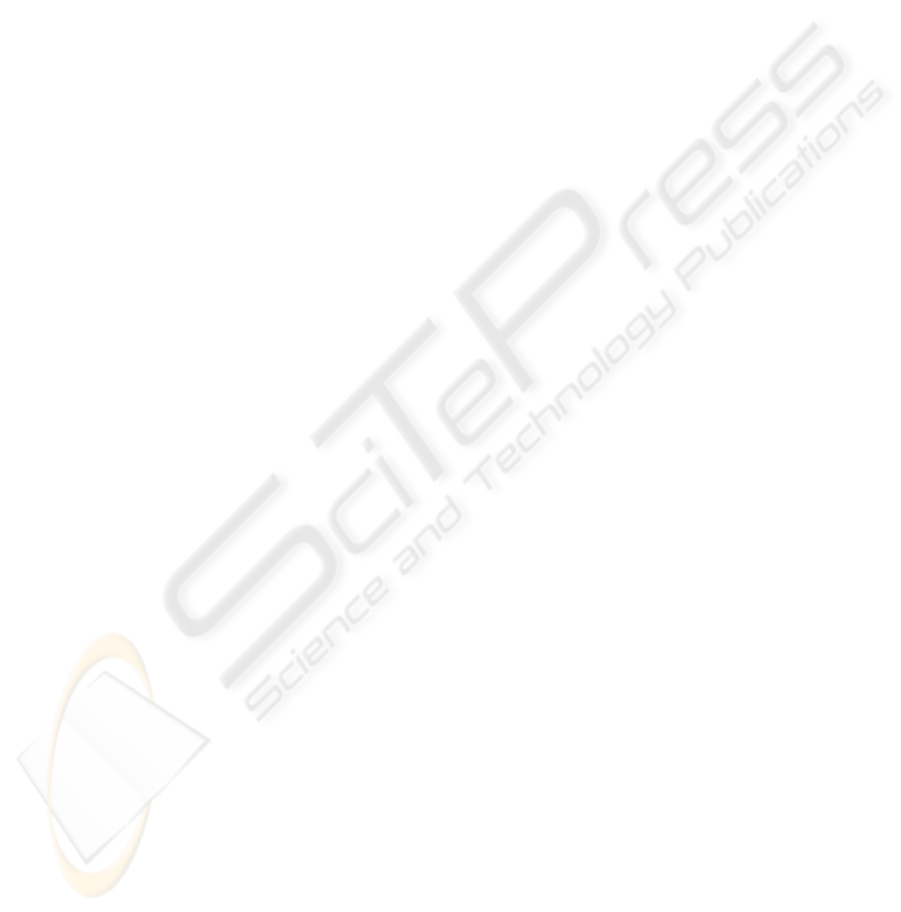
backbone are able to attract electrons from the
organic semiconductor, increasing the hole
concentration of PTAA than before DNA
immobilization (Zhang and Subramanian, 2007).
The increased in holes concentration would result in
the shift of V
T
to a lower negative voltage (positive
shift), causing an effective p-doping of PTAA.
After the hybridization with complementary
DNA, the threshold voltage (V
T
) shifted more
negatively (negative shift) to approximately -9 V.
Hybridization with complementary DNA would
result in double stranded DNA (dsDNA), where the
bases of two complementary single stranded DNA
(ssDNA) would pair up and form the double helix.
According to (Zhang and Subramanian, 2007),
dsDNA molecules could not be immobilized
(attached) to the organic semiconductor (PTAA) as
effectively as ssDNA molecules. This would result
in less interaction between DNA backbone and
PTAA that are known to be able to attract electrons,
lowering the holes concentration in PTAA and
hence, increasing the OFET’s V
T
to be more
negative. The low interaction ability between
dsDNA and PTAA is because the bases of DNA are
not exposed in dsDNA. The bases of DNA are
responsible to the hybrophobic interaction, resulting
in physical adsorption between ssDNA and PTAA,
i.e. immobilization of ssDNA.
4 CONCLUSIONS
The PTAA based p-channel OFETs were fabricated
successfully with a μmax of 0.25 cm
2
/Vs. The
amount of doping through the V
OX
in PTAA is
crucial in controlling the μ of the OFET. An
optimum V
OX
of 0.9 V was obtained, producing
OFET with the highest μ. A V
OX
> 1.0 V causes
degradation to the PTAA film, leading to poor
charge mobility, while V
OX
≤ 0.2 V does not
produce sufficient doping. These results
demonstrated that the μ of FET fabricated with
PTAA can be controlled by V
OX
and correlates with
the potential value of the cyclic voltammogram for
0.2 V ≤ V
OX
< 1.1 V. A preliminary DNA sensing
test was performed on the PTAA-OFET and a shift
in threshold voltage was observed after DNA
immobilisation and hybridisation, showing its
potential as DNA sensor. However, more detailed
study is required to realise this PTAA-OFET as
DNA sensor before it can be deployed.
REFERENCES
Chen, X., Inganas, O., 1996. Three-Step Redox in
Polythiophenes: Evidence from
Electrochemistry at an Ultramicroelectrode, The
Journal of Physical Chemistry 100 (37), 15202-15206.
Peng,H., Zhang, L., Spires, J. et al., 2007. Synthesis of a
functionalized polythiophene as an active substrate for
a label-free electrochemical genosensor, Polymer 48
(12), 3413-3419.
Roncali, J., 1992. Conjugated poly(thiophenes): Synthesis,
functionalization and applications, Chemical Reviews
92 (12), 711.
Tjitra Salim, N., Aw K.C., Peng, H. et. al., 2008. New 3-
((2':2'',5'':2'''-terthiophene)-3''-yl) acrylic acid as active
layer for organic field-effect transistor, Materials
Chemistry and Physics, 111 (1), 1-4.
Zhang, L., Peng, H., Kilmartin, P.A. et al., 2007.
Polymeric Acid Doped Polyaniline Nanotubes for
Oligonucleotide Sensors, Electroanalysis 19 (7-8),
870-875.
Zhang Q., Subramanian, V., 2007. DNA hybridization
detection with organic thin film transistors: Toward
fast and disposable DNA microarray chips, Biosensors
and Bioelectronics 22 (12), 3182-3187.
BIODEVICES 2010 - International Conference on Biomedical Electronics and Devices
162