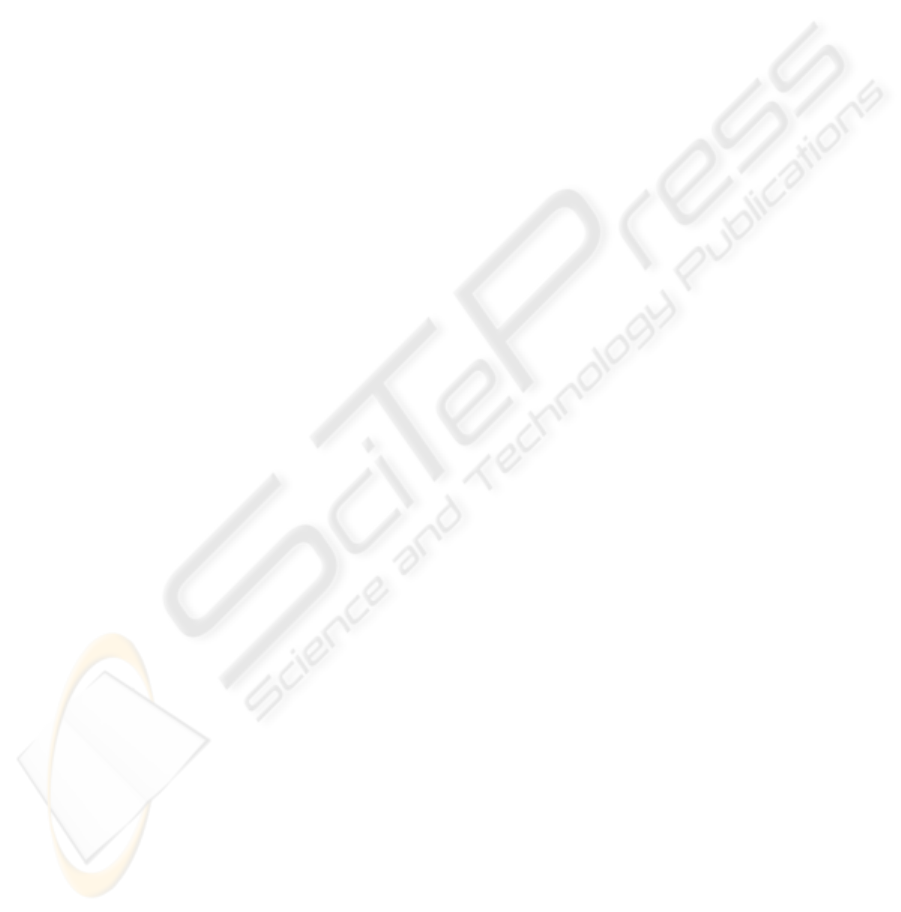
particles #1, #2, and #3 were compared with those of
the characteristic methyl group peaks in the pure
Iopamidol. In both methods, the efficiency of the
Iopamidol encapsulation increased dramatically in
particle #2 compared with particle #1 brought about
by the increase in the added crosslinker. The amount
of Iopamidol reached the maximum in particle #2
and decreased slightly in particle #3. Although the
degree of crosslinking detected by the
1
H NMR was
almost same in particles #2 and #3, the degree of
swelling in particle #2 was slightly higher. From this,
we can assume that particle #2 has a more flexible 3-
dimensional structure compared to particle #3,
which could lead to higher encapsulation of
Iopamidol in the former. The relation between the
degrees of crosslinking and swelling, as well as the
amount of Iopamidol encapsulations are graphically
summarized in Figure 5. Due to the presence of the
elastic force equilibrium between the retractive and
extended forces, the degrees of crosslinking and the
swelling exhibited opposite tendencies, while
Iopamidol reached the maximum amount at the
optimized encapsulation in particle #2.
6 CONCLUSIONS
The compact X-ray PIV system combining the
conventional X-ray radiography technique and PIV
velocity field measurement method was developed.
Through preliminary tests, the spatial and temporal
resolution of this system was found to be higher than
any conventional clinical instruments. In addition,
new X-ray flow tracers were fabricated by
encapsulating Iopamidol into bio-compatible
polymer PVA. The functional characteristics of the
fabricated microparticles were checked. With
increasing the amount of crosslinker, the degree of
crosslinking and the efficiency of the Iopamidol
encapsulation were increased. In near future, the
developed system would be employed usefully for
measuring in vivo velocity field information of blood
flows.
ACKNOWLEDGEMENTS
This work was supported by the Creative Research
Initiatives (Diagnosis of Biofluid Flow Phenomena
and Biomimic Research) of MEST/KOSEF.
REFERENCES
Adrian, R. J. (1991) “Particle-imaging techniques for
experimental fluid mechanics”, Annual Review of
Fluid Mechanics, Vol. 23, pp. 261-304.
Fouras, A., Dusting, J., Lewis, R. and Hourigan, K. (2007)
“Three-dimensional synchrotron X-ray particle image
velocimetry”, Journal of Applied Physics, Vol. 102,
064916.
Im, K.S., Fezzaa, K., Wang, Y. J., Liu, X., Wang, J. and
Lai, M. C. (2007) “Particle tracking velocimetry using
fast X-ray phase-contrast imaging”, Applied Physics
Letters, Vol. 90, 091919.
Jenneson, P. M., Gilboy, W. B., Morton, E. J. and Gregory,
P. J. (2003) “An-ray micro-tomography system
optimised for the low-dose study of living organisms”,
Applied radiation and isotopes, Vol. 58, pp. 177-181.
Kim, G. B. and Lee, S. J. (2006) “X-ray PIV
measurements of blood flows without tracer particles”,
Experiments in Fluids, Vol. 41, pp. 195-200.
Lee, S. J. and Kim, G. B. (2003) “X-ray Particle Image
Velocimetry for measuring quantitative flow
information inside opaque objects”, Journal of Applied
Physics, Vo. 94, pp. 3620-3623.
Lee, S. J. and Kim, S. (2005) “Simultaneous measurement
of size and velocity of microbubbles moving in an
opaque tube using an X-ray particle tracking
velocimetry technique”, Experiments in Fluids, Vol.
39, pp. 492-497.
Lee, S. J. and Kim, Y. M. (2008) “In vivo Visualization of
the Water-refilling Process in Xylem Vessels Using X-
ray Micro-imaging”, Annals of Botany, Vol. 101, pp.
595-602.
Malek, A., Alper, S. and Izumo, S. (1999) “Hemodynamic
shear stress and its role in atherosclerosis”, JAMA, Vol.
282, pp. 2035-2042.
Matsumura, S., Kurita, H. and Shimokobe, H. (1993)
“Anaerobic biodegradability of polyvinyl alcohol”,
Biotechnology Letters, Vol. 15, pp. 749-754.
Matsumura, S., Takahashi, J., Maeda, S. and Yoshikawa,
S. (1988) “Molecular design of biodegradable
functional polymers, 1. Poly(sodium vinyloxyacetate)”,
Die Makromolekulare Chemie, Rapid
Communications, Vol. 9, pp. 1-5.
Nord, F. F. (1936) “Dehydrogenation activity of Fusarium
lini B”, Naturwissenschaften, Vol. 24, 763.
Pahernik, S., Griebel, J., Botzlar, A., Gneiting, T., Brandl,
M., Dellian, M. and Goetz, A.E. (2001) “Quantitative
imaging of tumour blood flow by contrast-enhanced
magnetic resonance imaging”, British Journal of
Cancer, Vol. 85, pp. 1655-1663.
Rhode K.S., Lambrou T., Hawkes D.J. and Seifalian A.M.
(2005) “Novel approaches to the measurement of
arterial blood flow from dynamic digital x-ray images”,
IEEE TRANSACTIONS ON MEDICAL IMAGING,
Vol. 24, pp. 500-513.
DEVELOPMENT OF A COMPACT X-RAY PIV SYSTEM AND NEW X-RAY FLOW TRACERS - Visualization of
Opaque Biofluid Flows using X-ray PIV System
37