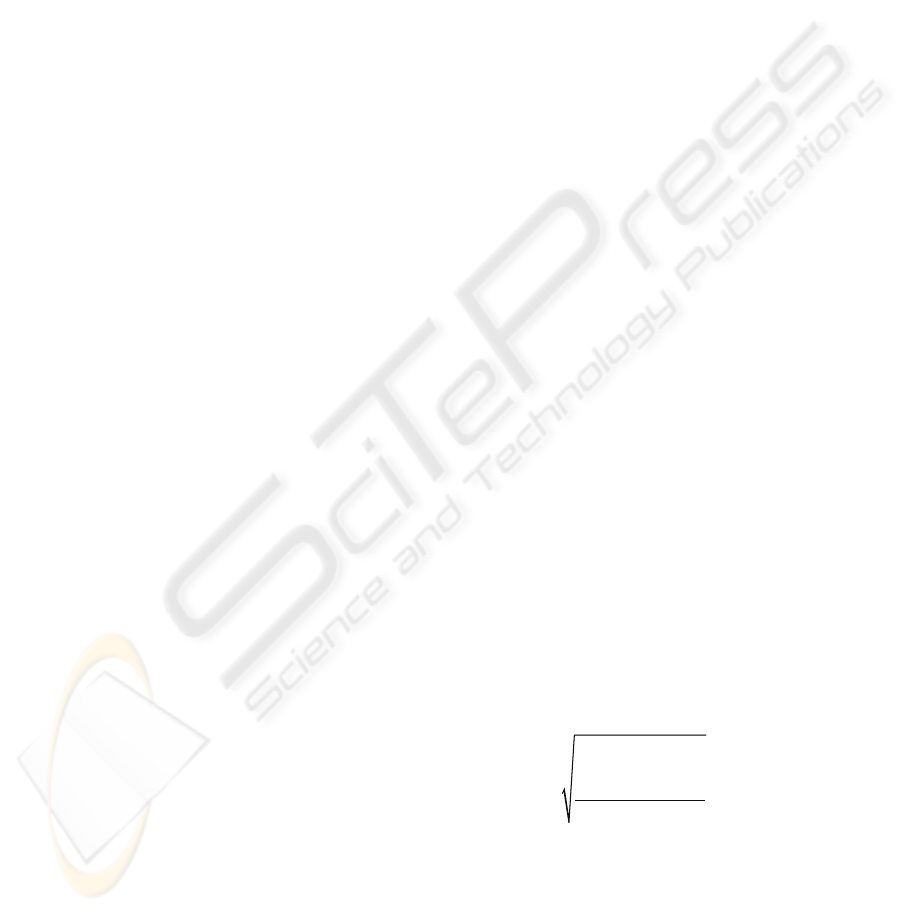
found that, in work environments, these methods are
complicated to set up and can only easily be applied
in laboratory. On the other hand, electronic sensors
able to provide orientation based on accelerometers
and gyroscopes have been developed and applied to
solve the relevant outdoor application problems of
the image-based methods. These sensors have been
used and evaluated for the 3D measurement of trunk,
lower and upper segments, during posture, walking
and rising from a chair, in both normal and
pathological conditions (Pfau, 2005, Lau, 2008,
Plamondon, 2007, Coley, 2007, Veltink, 2007,
Boonstra, 2006, Zijlstra, 2008).
In the present study we compared the angular
behaviour of the pelvis in the sagittal, frontal and
horizontal plane calculated with a wearable inertial
device including triaxial accelerometers and triaxial
gyroscopes with that computed with a high precision
and accuracy optoelectronic motion analysis system.
We recorded the angle trajectories and excursions
during the following tasks: standing anterior
reaching and grasping, standing oblique reaching
and grasping, standing oblique opposite reaching
and grasping, anterior trunk flexion and sit to stand.
The assessment of pelvis during posture and
movement is important in improving our
understanding of the motor strategies at work and
preventing injuries (i.e. low back pain) and
mechanical whole body fatigue.
2 MATERIALS AND METHODS
Ten healthy male subjects (mean age 38 ± 4 years,
range 20-55 years) were enrolled. All gave their
written informed consent after receiving a full
explanation of the study, which conformed to the
requirements of the Declaration of Helsinki.
We used a Wi-Fi transmission miniaturized
device integrating an accelerometer and a gyroscope
(MicroStrain 3DM-GX2, MicroStrain, Inc.,
Williston, USA) placed directly on the skin over the
sacrum. The device offers a range of output data
quantities from fully calibrated inertial
measurements to computed orientation estimates.
All quantities are fully temperature compensated and
corrected for sensor misalignment. The angular rate
quantities are further corrected for G-sensitivity and
scale factor non-linearity to third order.
The extracted curves and the calculated angles
were compared to those simultaneously acquired by
a high-quality optical motion analysis system
(SMART-E System, BTS, Milan, Italy, Ferrigno and
Pedotti 1985) consisting of eight infra-red ray
cameras (operating at 120 fps) to detect the
movements in three-dimensional space of three
retro-reflective markers placed on the skin over the
sacrum and the right and left anterior superior iliac
spinae. Data processing was performed using
Analyzer software (BTS, Milan, Italy).
Before starting formal measurements, all
subjects did a practice session to familiarize
themselves with the experimental procedure and
with the tasks consisting of eleven movements
performed in a quiet room with normal indoor
temperature and lighting. The performed tasks were:
standing anterior reaching and grasping, standing
oblique reaching and grasping, standing oblique
opposite reaching and grasping, anterior trunk
flexion and sit to stand. Standing reaching and
grasping tasks have been performed with the subject
starting from a standing posture, with the trunk kept
upright, left and right arm lying alongside the body
and performing the movement, in a natural fashion.
In the anterior reaching and grasping the subjects
picked up, with the right hand, a cylinder (diameter,
3 cm; height, 6 cm; weight, 300 g) positioned on a
shelf in line (on the anterior direction) and at the
same height of the right shoulder, and returned the
cylinder to the starting position. The oblique and the
oblique opposite reaching and grasping tasks were
performed in the same manner of the anterior
reaching and grasping but with the object positioned
at ±45° with respect to the anterior direction. The
anterior trunk flexion was performed through a
maximal anterior flexion of the trunk. In the sit to
stand movement the subjects were seated
comfortably on a chair and got stand up in a natural
manner and at their preferred velocity. Ten cycles
were recorded for task and each person. Angular
excursion data were normalized to the movement
duration and reduced to 100 samples. For the
comparison of the Range of Motions (ROMs) we
used the root mean squared error (RMS):
()
2
,,
11
N
oi di
xx
RMS
n
=
−
=
∑
(1)
whereas the Coefficient of Multiple Correlation
(CMC), i.e. the positive square root of the adjusted
coefficient of multiple determination (Kabada et al.
1989, Steinwender et al. 2000) by means of the
following formula:
GLOBAL BIOMECHANICAL EVALUATION DURING WORK AND DAILY-LIFE ACTIVITIES
109