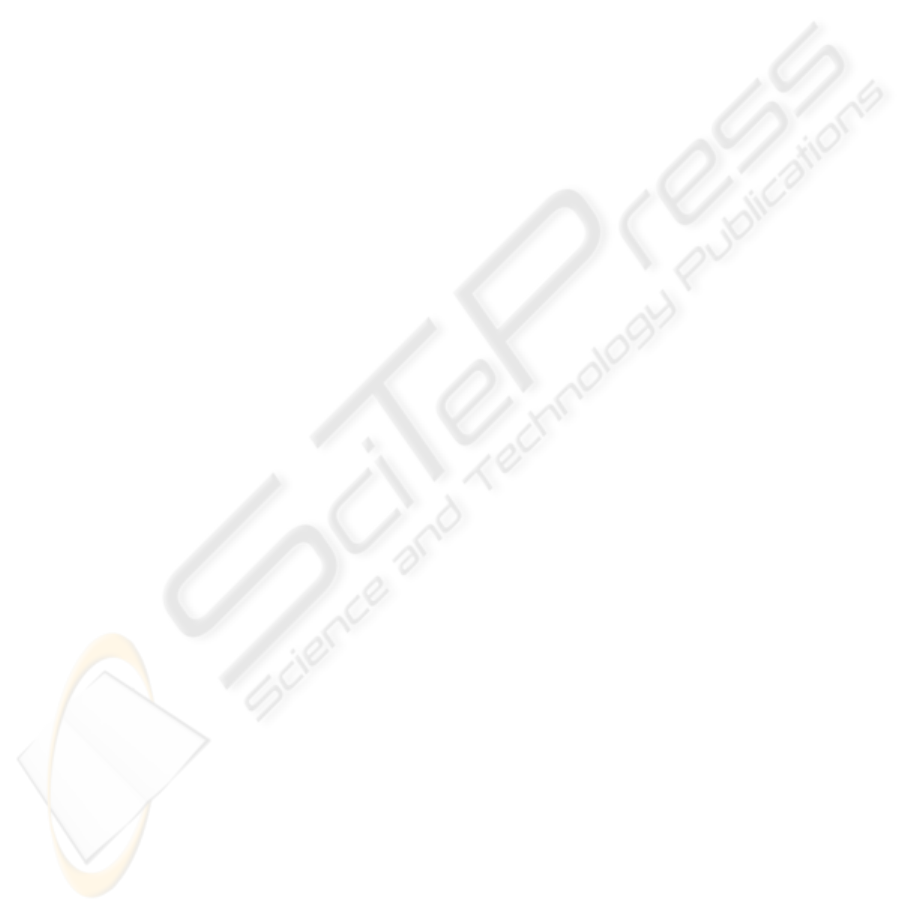
DETECTION OF DNA AT THE MICROCHIP
Study of DNA Detection Microchip by Oxidation Peak of DNA
Gi-sung Joo, You-Cheol Jang, Islam Atm Kamrul
Department of Nano Science and Engineering, Myongji University, Gyeonggi, Korea
Yong-Sang Kim
Department of Electric Engineering, Myongji University, Gyeonggi, Korea
Keywords: Capillary electrophoresis, Amperometry, Polyacrylamide, Microfluidics.
Abstract: We have demonstrated the separation and detection of DNA on the microchip based on amperometric with
capillary electrophoresis. To enhance analytic perfermance such as throughput and analysis time,
electrophoretic separation of DNA in capillaries or on microchips has been investigated using various
microchip structures. Compared with commonly used laser induced fluorescence method, this method is
more compatible with microchip and offer improved portability and miniaturization. Through Cyclic
voltammetric experimental, we could optimized detection voltage for detection of DNA. At the optimal
detection voltage, DNA fragments were successfully separated and detected with high sensitivity and stable
baseline.
1 INTRODUCTION
DNA analysis is important step in area of
biochemistry as well as molecular biology. In most
cases DNA is detected by fluorescent or optical
spectroscopy after agarose gel electrophoresis.
However, this procedure is tedious, time consuming
and require expensive equipments. Therefore
miniaturization of DNA analysis is necessary.
Capillary electrophoresis (CE) microchip, intro-
duced by Manz et al. in the early 1990s, alternative
for separation of biological compounds. To enhance
throughput and to shorten analysis time, electro-
phoretic separation of DNA in capillaries or on
microchips has been suggested using different
microchip structures. Optical microarrays are
arguably the most widely used type of biosensors in
DNA analysis where detection of specific DNA
sequences are based on labeling sample DNAs with
fluorophores. While fluorescence-based detection
technologies have shown tremendous utility, they
suffer from the drawbacks of labor-intensive sample
preparation, high cost, and complex and bulky
fluorescence detection instrumentation. However,
amperometric method is measured to change current
according to oxidation of analytes. Guanine and
adenine electro-oxidation is useful for the
amperometric method. Using this technique,
fragments of negatively charged DNA can be
resolved inside a capillary by application of potential.
The resolved DNA can be detected ampero-
metrically using oxidation peak of adenine base.
In the present work, we have attempted to develop
an amperometric. The microchips are usually
fabricated from silicon and glass. However,
polymeric materials are also used due to their
properties such as low cost, high flexibility, and
simply fabrication procedures. Several polymers
such as poly(dimethylsiloxane) (PDMS) and poly
(methyl methacrylate) (PMMA), polyester have
been reported for fabrication of microchip. Our
microchip was fabricated on glass substrate and
microchannels were laid in PDMS mold. The
capillary was filled with polyacrylamide gel and
separation was achieved by application of DC
potential. This technique was used in resolving DNA
fragments
117
Joo G., Jang Y., Atm Kamrul I. and Kim Y. (2010).
DETECTION OF DNA AT THE MICROCHIP - Study of DNA Detection Microchip by Oxidation Peak of DNA.
In Proceedings of the Third International Conference on Biomedical Electronics and Devices, pages 117-120
DOI: 10.5220/0002744001170120
Copyright
c
SciTePress