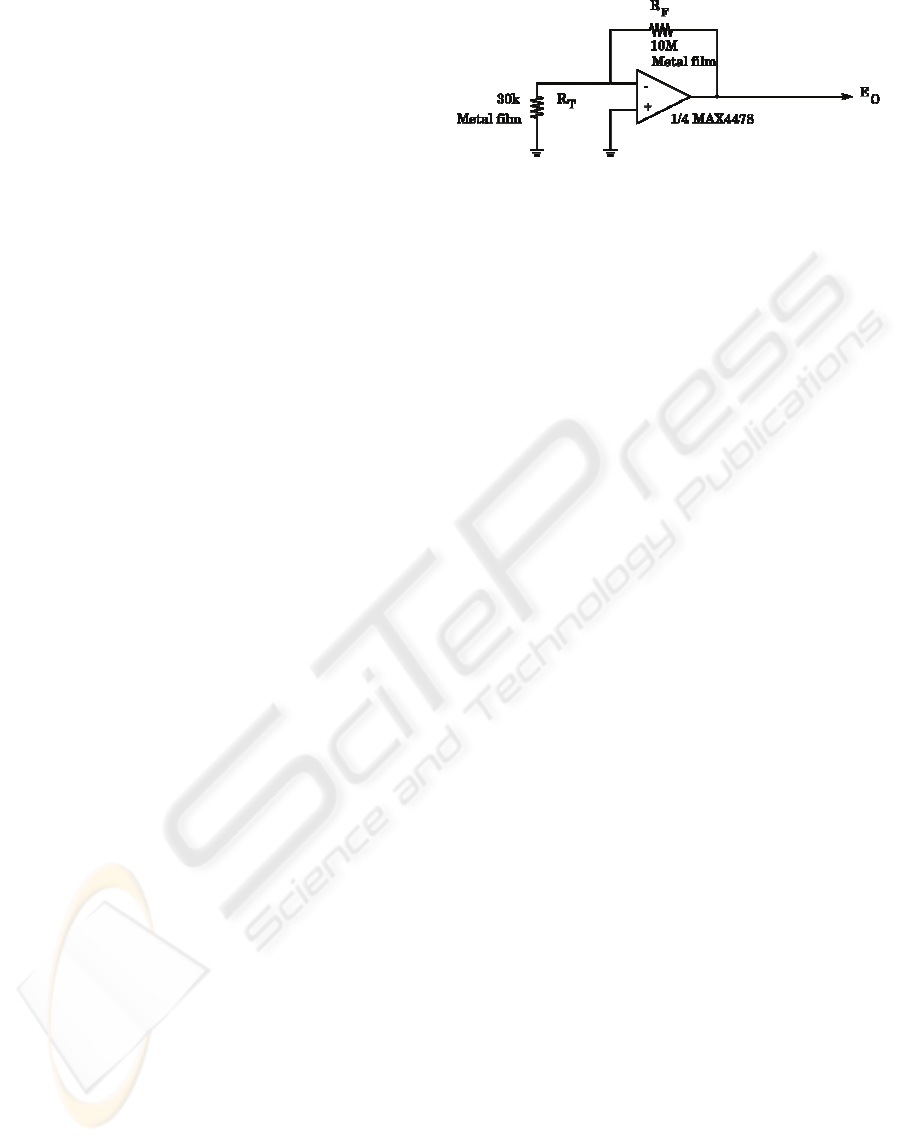
We cannot reverse bias the IR photodiodes due
to their high reverse saturation current. Increasing
reverse bias increases the dark current and shot noise
on the dark current would dominate other noise
sources and reduce the SNR. Also, since the dark
current increases with more reverse bias as the
photocurrent remains constant, a smaller portion of
the current flowing into the TIA is due to the
photocurrent, reducing the SNR.
2.3 Circuit Design
Figure 5 shows the final circuit design used for each
channel. We added the shot noise on the offset
current, op amp input current noise, and op amp
voltage noise for a number of op amps to select an
op amp for the TIA. Here the offset current is the
sum of the op amp input offset current and the
current in R
T
due to the op amp offset voltage. We
included shot noise on the offset current since the
source of the photodiode current is a diode junction.
We chose the MAX4478 (Maxim, 2005) because it
minimized the sum of these noise voltages.
The chief factors affecting choice of the ADC
are the resolution, number of delta-sigma (∆-Σ)
blocks in the device, ability to daisy-chain serial data
ports of devices, and conversion time. We chose a
24-bit ∆-Σ ADC with 8 channels, 8 ∆-Σ blocks, and
maximum sampling rate of 52.7 kHz when using
high resolution (Texas Instruments, 2008). Four
ADCs are necessary to sample all 32 channels
simultaneously. We need a minimum of 20 bit ADC
resolution to keep the quantization error well below
the system noise. Since there is one ∆-Σ block for
each photodiode channel, multiplexing ADCs is
unnecessary, allowing time efficiency and
simplifying the software required to archive data.
The serial ports of the ADC we chose can be
daisy-chained which also simplifies the system
because only one serial port is required. We need to
record four types of samples to calculate the glucose
concentration: with LED 1 on/LED2 off, both LEDs
off, LED1 off/LED2 on, and both LEDs off. Since
we sample all channels simultaneously and typically
record N = 250 samples per second, recording four
types of data samples requires a sampling rate of 1
Figure 5: Schematic of one DAU channel.
kHz, well below the ADS1278 maximum sample
rate. We selected a low noise voltage reference with
noise voltage of 1.5 μV and a low temperature
coefficient of 0.6 PPM/deg. C. (Cirrus Logic, 2009)
3 EXPERIMENT AND RESULTS
Figure 6 shows the method we use to record
experimental data. The time per data point, T
Data
, is 1
second and N = 250 so that T
Sample
= 1/250 second.
The DAU samples the voltage on all channels at
points labeled S
n
. At points labeled D
m
the average
value of the samples for all channels are calculated
and archived. When we use the glucose sensor the
four types of data mentioned above must be
recorded in each sample period to calculate the
glucose concentration. While verifying the SNR of
the DAU system in this paper we utilize low noise
resistors and only one of the four types of data is
required since there are no LEDs present without the
glucose sensor.
To test the SNR we record a large (1k data
points) set of experimental data and calculate the
SNR. Figure 7 shows a plot of channel voltage vs.
time for a representative channel. Figure 7 shows
2000 data points from channel 1 taken at a rate of 1
Hz – the last 1000 data points were used to calculate
the SNR. The plot shows approximately 20 µV of
drift with +/- 10 µV of noise in the last 1000 data
points. The standard deviation of this portion of data
is 9.12 μV and the SNR for channel 1 is calculated
to be 40.4 dB. Fig. 8 shows the SNR for all 32
channels. The mean SNR is 41.7 dB and the
standard deviation of the SNR is 2.0 dB. Channels
15 and 31 do have 5 to 7 dB more noise than the
other channels. This is likely due to slight
differences in noise characteristics of the op amps.
Fig. 4 also shows the thermal noise present in the
BIODEVICES 2010 - International Conference on Biomedical Electronics and Devices
198