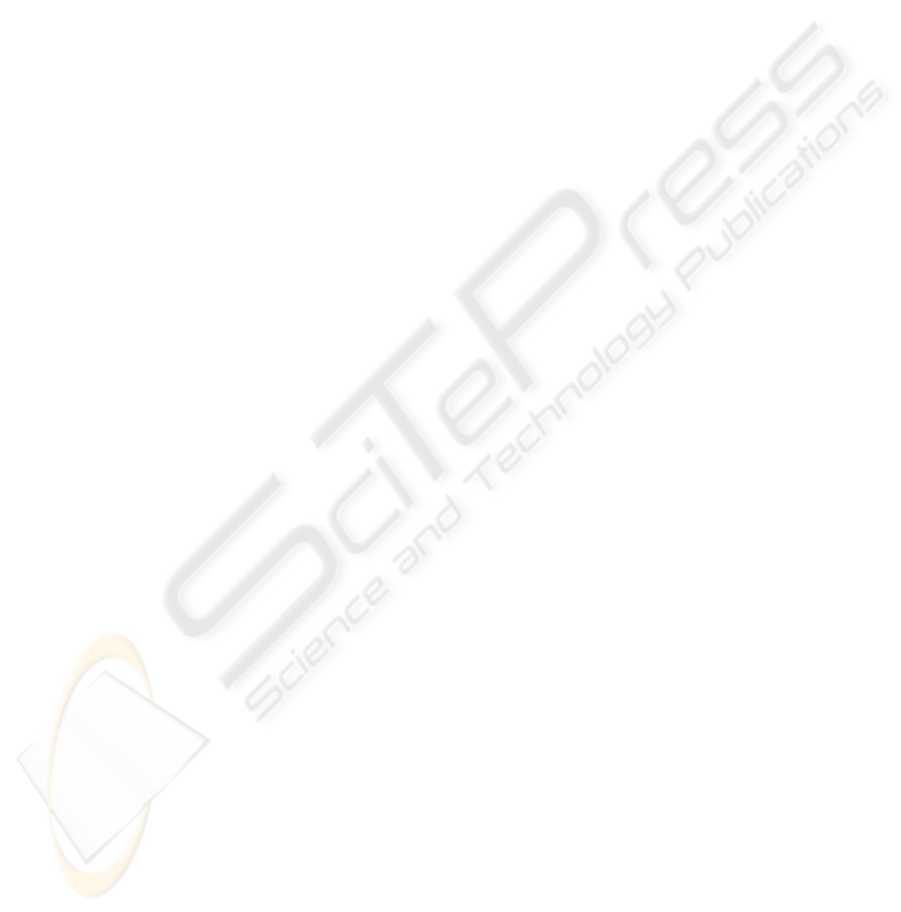
To the best of our knowledge, the smart ligament
balancer proposed in this study is the first one that
could quantitatively assess the mediolateral balance
of collateral ligament intra- and postoperatively in
both extension and flexion. In addition, this balancer
could allow a continuous assessment of ligament
imbalance over the whole range of flexion while
most balancing instruments assess this balance at
full extension and 90° of flexion and don’t allow
measurements at other positions of flexion (Attfield,
1996).
Since the medial and lateral collateral ligaments
of knee are of different cross-section, length and
shape and do not represent extensile strings, but
viscoelastic, extendible structures, the ligament
imbalance could not be constant at different
separation gaps and depends on the compressive
tension used to distract the bones. Therefore,
ligament imbalance must be quantified at different
distraction gaps by tensing the knee by equal forces
both medially and laterally but with different force
at each time. This is completely possible by means
of our smart knee balancer given that the ligament
imbalance is quantified by measuring the difference
in height between the medial and lateral sides of a
trapezoidal tibiofemoral gap produced when
identical tensions are applied to both medial and
lateral ligaments of the knee. In addition, the
parallelism of the upper trays is perfectly ensured by
the use of scissor mechanism instead of inflatable
rubber bladders (Marmignon, 2004). Furthermore,
the continuous movement of the upper tray ensures a
continuous augmentation of the gap height rather
than the discrete movement achieved by inserting
spacer blocks (Crottet, 2005), which decreases the
time and complexity of knee surgery.
REFERENCES
Attfield, S. F., Warren-Forward, M., Wilton,
T., Sambatakakis, A., 1994. Measurement of soft
tissue imbalance in total knee arthroplasty using
electronic instrumentation, Medical Engineering and
Physics, 16(6):501–505.
Attfield, S. F., Wilton, T. J., Pratt, D. J., Sambatakakis A.,
1996. Soft tissue balance and recovery of
proprioception after total knee replacement, Bone and
Joint Surgery, 78(4):540–545.
Crottet, D., Maeder, T., Fritschy, D., Bleuler, H., Nolte,
L.P., Pappas, I.P., 2005. Development of a force
amplitude- and location-sensing device designed to
improve the ligament balancing procedure in TKA,
IEEE Transactions on Biomedical Engineering,
52(9):1609–1611.
Fehring, T. K., Valadie, A. L., 1994. Knee instability after
total knee arthroplasty, Clinical Orthopaedics and
Related Research, 299:157–162.
Griffin, F., Insall, J., Scuderi, G., 2000. Accuracy of soft
tissue balancing in total knee arthroplasty,
Arthroplasty, 15(8):970–973.
Insall, J.N., 1984. Surgery of the Knee, Chapter: Total
knee replacement, Churchill Livingstone, 1
st
edition.
Insall, J.N., 1993. Surgery of the Knee, Chapter: Surgical
techniques and instrumentation in total knee
arthroplasty, Churchill Livingstone. 2
nd
edition.
Laskin, R.S., Riegèr, M.A., 1989. The surgical technique
for performing a total knee replacement arthroplasty,
The Orthopedic Clinics of North America, 20(1):31–
48.
Marmignon, C., Leimnei, C., Cinquin, P., 2004. Robotized
distraction device for knee replacement surgery,
Computer Assisted Radiology and Surgery, 1268:638–
643.
BIODEVICES 2010 - International Conference on Biomedical Electronics and Devices
142