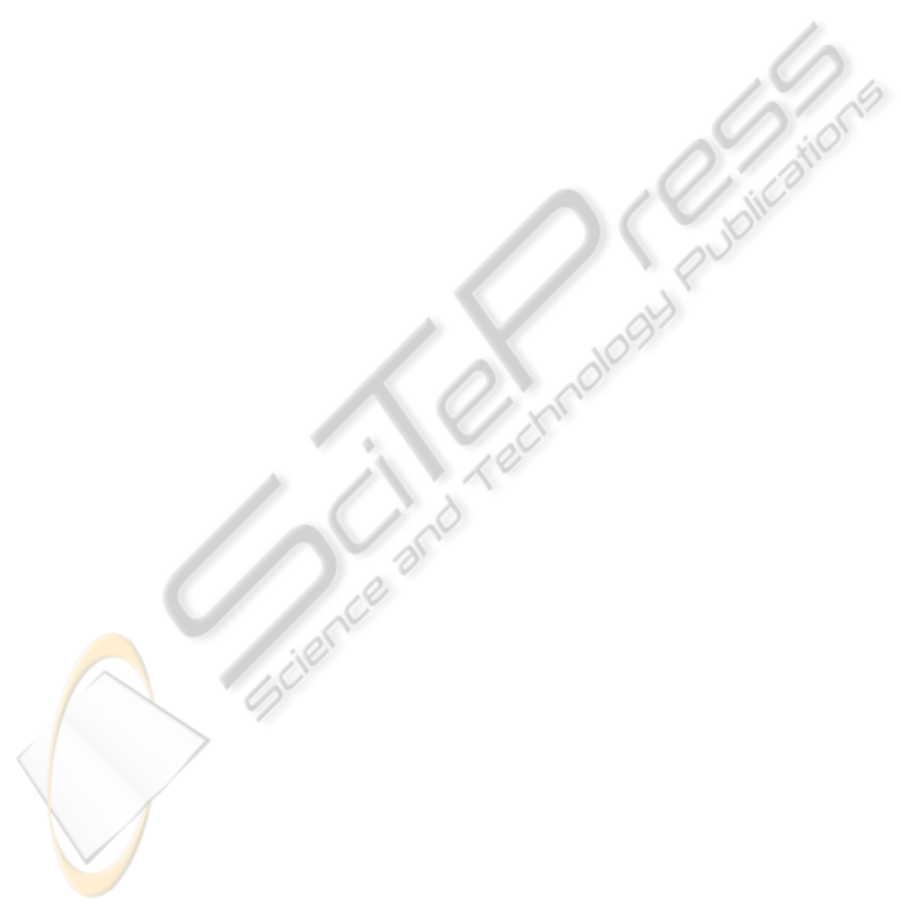
On the other hand, the presence of disturbances
on power distribution also affect the energy efficiency
of the system. As far as energy efficiency is con-
cerned in a power distribution system, the two dom-
inant factors in PQ are its unbalanced and harmonic
distortion. In an electrical installation when single-
phase loads (especially those with non-linear charac-
teristics), are not evenly and reasonably distributed
among the three-phases of the supply, we are in the
presence of unbalance. Voltage unbalance in a three-
phase system causes three-phase motors to draw un-
balanced current. This phenomenon causes additional
power losses in conductors and motors and can cause
the rotor of a motor to overheat.
Among all categories of electrical disturbances,
the voltage sag (dip) and momentary interruption are
the nemeses of the automated industrial processes.
Voltage sag is commonly defined as any low voltage
event between 10 and 90% of the nominal RMS volt-
age lasting between 0.5 and 60 cycles. Momentary
voltage interruption is any low-voltage event of less
than 10% of the nominal RMS voltage lasting be-
tween 0.5 cycles and 3 seconds. In medium voltage
distribution networks, voltage sags are mainly caused
by power system faults. Fault occurrences elsewhere
can generate voltage sags affecting consumers differ-
ently according to their location in the electrical net-
work. Even though the load current is small compared
to the fault current, the changes in load current dur-
ing and after the fault strongly influence the voltage
at the equipment terminals. It has been discovered
that the 85% of power supply malfunctions attributed
to poor PQ are caused by voltage sag or interruptions
of fewer than one second duration. Starting large mo-
tors can also generate voltage sags, although usually
not so severe. In comparison with interruptions, volt-
age sags affect a larger number of customers and for
some customers voltage sags may cause extremely se-
rious problems. These can create problems to sen-
sitive equipment if it is designed to operate within
narrow voltage limits, or it does not have adequate
ride-through capabilities to filter out fluctuations in
the electrical supply.
Over-voltage is an RMS increase in the AC volt-
age, at the power frequency, for durations greater than
a few seconds, and can be the result of a programmed
utility operation, or the effect of an external eventu-
ality (IE3, 1995a). Under normal operating condi-
tions, the steady-state voltage is regulated by the util-
ity within a limits band accepted by the EN-50160.
Deviations from these limits are rare, and the utility
can actuate readily to correct them, if known their oc-
currence, by acting on conventional distribution tech-
nologies, such as tap-changing transformers (Moreno
et al., 2007).
However, under the typical operating conditions
of a power system there is risk of damaging due to
a momentary excess of voltage. Although by them-
selves they would be described as ”abnormal”, it is
possible to distinguish between surges and swells. A
surge is an over-voltage that can reach thousands of
volts, lasting less than one cycle of the power fre-
quency, that is, less than 16 milliseconds. A swell
is longer, up to a few seconds, but does not exceed
about twice the normal line voltage.
Power system surges, based on waveform shapes,
can be classified into ”oscillatory transients” and ”im-
pulsive transients” (IE3, 1995b; Paul, 2001) and they
are the goal of the present research work. Oscilla-
tory transient surges show a damped oscillation with
a frequency range from 400 Hz to 5 kHz or more. Im-
pulsive transient surges present a fast rise time in the
order of 1 ns-10 µs over the steady state condition of
voltage, current or both, that is unidirectional in po-
larity (primarily either positive or negative), reaching
hardly twice the peak amplitude of the signal. They
are damped quickly, presenting a frequency range
from 4 kHz to 5 MHz, occasionally reaching 30 MHz.
Categorization of electrical transients based on
waveform shapes and their underlying causes (or
events) has been studied in (Bollen et al., 2005), and
a few previous studies (De la Rosa et al., 2007;
¨
Omer
Nezih Gerek and Ece, 2006, ) using HOS for feature
extraction of electrical signals have shown the pos-
sibility of distinguish transients based on details be-
yond the second-order. In a real-life 50-Hz power
line signal, it is very common to find these transients.
In Fig. 1 we show an example of anomalous signal,
including transients which are not classified between
short-duration and long-duration. We show the com-
putation of three higher-order time-domain statistics
in order to introduce them qualitatively. The second-
order estimator operates as an increase-of-power de-
tector, showing the bumps associated to the increase
of power, which in turn are associated to the anoma-
lies of the power-line sine wave, but the third and
fourth-order sliding cumulants have to be interpreted
further. The most intuitive procedure is to calculate
their maxima and minima.
Once the foundations of PQ have been settled
down, in the following Section we present higher-
order statistics in the time-domain in order to present
the signal processing tool, along with a basic example
which shows the performance of the statistical esti-
mators which have been used in the computation of
the cumulants. This example also motivates the use
of HOS in time-series characterization.
CLASSIFICATION OF POWER QUALITY DISTURBANCES VIA HIGHER-ORDER STATISTICS AND
SELF-ORGANIZING NEURAL NETWORKS
185