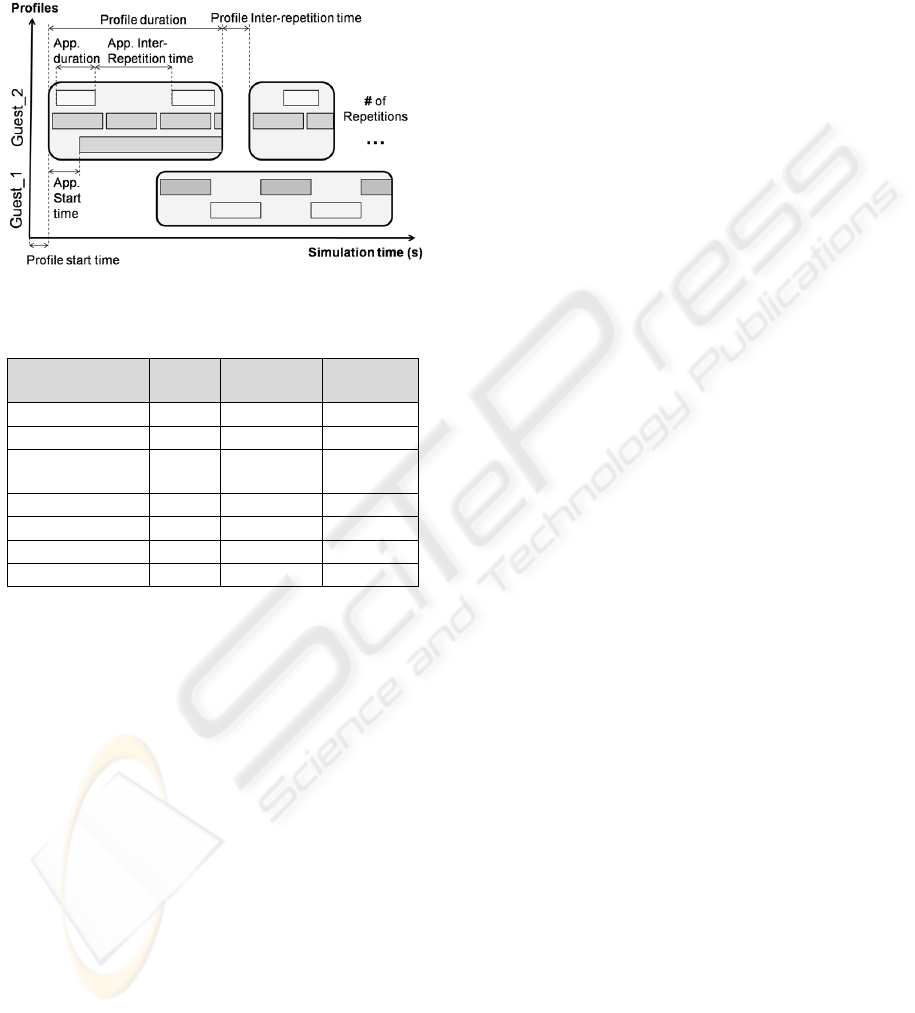
performed daytime simulations to see the dynamic
evolution of traffic as users connect their devices
into the network, and to obtain peak bandwidth
demands, which will be dominated by the heavy
video traffic. As daily TV usage begins early in the
Figure 4: Guest profile definition.
Table 3: Application profile definition.
Application
BW
(Mb/s)
Duration (s)
Repetition
(s)
Web browsing* 1-100 Exp(600) Exp(600)
Email 1 Exp(60) Exp(600)
Database access 0.5 Uni(300) Uni(300-
600)
VoIP 0.096 Exp(600) Exp(1800)
Videoconference 4 Exp(600) Exp(7200)
File transfer 100 Exp(300) Exp(1800)
IPTV 6-24 Exp(7200) Exp(21600)
* Includes also video browsing, social networking and immersive online
games and environments.
morning, we can see in Figure 5 the bandwidth
demands per floor, quickly over passing the Fast
Ethernet limit of 100 Mb/s. Making use of Gigabit
Ethernet seems mandatory to avoid congestion if
intensive video traffic (HDTV or high resolution
videoconferencing) is considered. This is specially
important in tele-healthcare applications. The
similarity among floors in the bandwidth traces over
the random statistical variability is due to the
identical guest profiling used per floor to simplify
design and simulation time and future work intends
to add further detail to such guest distribution.
As a way to measure the responsiveness of the
system, we we will use the channel switching time
of the hospitality IPTV system. The channel
switching time (or zapping delay) can be defined as
the time difference between the user asking for a
channel change by pressing a button on the remote
control and the display of the first frame of the
requested channel on the TV screen. In analog TV,
channel change is around 100 ms since it only
involves the receiver tuning to a new carrier
frequency, demodulating the analog signal and
displaying the picture on the screen. IPTV channel
switching times can be higher due to delay factors
(Uzunalioglu, 2009), like digital video
decompression and buffering, IP network related
issues (frame encapsulation, IGMP group joining,
congestion, etc.) and content management (paid
subscription channels, parental filtering, etc.). Fast
switching IPTV systems are expected to have
zapping delays of less than a second. To make our
study more general, we will only consider the
network components of the channel switching delay,
as video codification and content management
greatly depends on the specific IPTV
implementation.
In Figure 6 we see the influence of full HDTV
data streams (12 Mb/s per channel) over the channel
switch requests (the zapping delay). We observe
that, under a gigabit Ethernet network, this value
still falls far from the margin of 125 ms limit for
seamless zapping time, but over slower connections,
delay quickly builds up and can degrade user
experience and congest the remaining data network.
For the same situation, but considering all users
streaming high-definition WebTV video (6.3 Mb/s)
instead of IPTV, or high-definition
videoconferencing (4.3 Mb/s), there would be an
average frame delivery delay of 2.74 ms under a
Fast Ethernet network, or only 0.38 µs under a
Gigabit Ethernet one.
We can conclude that the presented architecture
remains valid for the services evaluated and still
holds enough margins to cope with spikes due to
seasonal trade show attendance or an increase in
holiday travelling. Moreover, dynamic network load
balancing on the optical domain can spread future
demands over all available physical media, avoiding
traffic surges, server bottlenecks, connectivity losses
and downtimes. Considering specially the RoF
distribution system, more wireless and mobile
capacity can be allocated to an area (e.g. conference
hall) during peak times and then re-allocated to other
areas when off-peak (e.g. guestrooms in the
evenings). This obviates the requirement for
allocating permanent capacity, which would be a
waste of resources in cases where traffic loads vary
frequently and by large margins. Future explorations
on the hospitality scenario will include the dynamic
use of different optical wavelengths through
reconfigurable Wavelength Division Multiplexing
(WDM).
DCNET 2010 - International Conference on Data Communication Networking
144