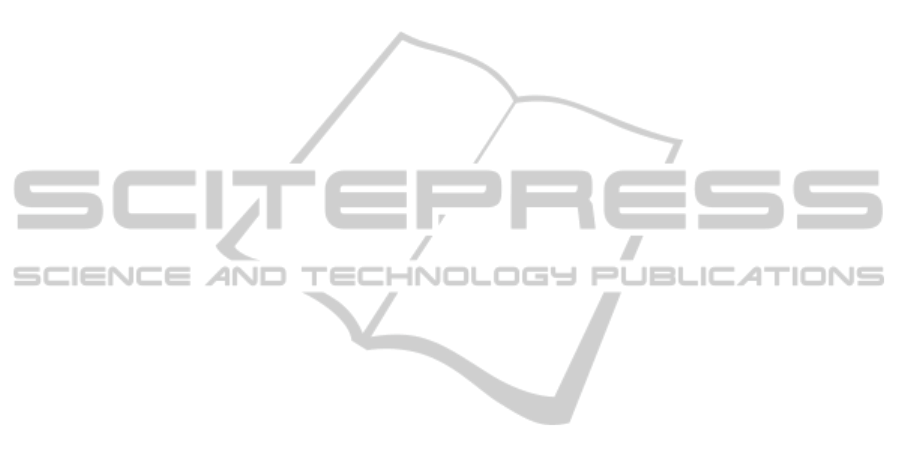
Demmler-Harrisson G. L., 2009, Congenital
cytomegalovirus: public health action towards
awareness, prevention, and treatment, J. of Clin.
Virol., Vol. 46 Suppl 4, pp. S1-5.
Fowler K. B., Dahle A. J., Boppana S. B., Pass R. F.,
Newborn hearing screening: will children with hearing
loss caused by congenital cytomegalovirus infection
be missed?, 1999, J. Pediatr. Vol.135, pp. 60-64.
Huikko K., Kostiainen R., Kotiaho T., Introduction to
micro-analytical systems: bioanalytical and
pharmaceutical applications, 2003, Eur. J. of
Parmaceutical Sciences, Vol.20, pp.149-171.
Kardous F., Simon B., Yahiaoui R., Manceau J. F.,
Boireau W., Improving immunosensor performances
using acoustic mixer on droplet microarray, 2010,
Biosens. And Bioelec. In Press,
doi:10.1016/j.bios.2010.09.007
Lazzarotto T., Varani S., Guerra B., Nicolosi A., Lanari
M., Landini M. P., Prenatal indicators of congenital
cytomegalovirus infection, 2000, J. Pediatr. Vol.137,
pp. 90–95.
Liao C. S., Lee G. B., Liu H. S., Hsieh T. M., Luo C. H.,
Miniature RT-PCR system for diagnosis of RNA-
based viruses, 2005, Nucleic Acids Res. Vol. 33, pp.
e156.
Nigro G., Mazzocco M., Anceschi M. M., La Torre R.,
Antonelli G., Cosmi E. V., Prenatal diagnosis of fetal
cytomegalovirus infection after primary or recurrent
maternal infection, 1999, Obstet. Gynecol. Vol.94, pp.
909-914.
Park J. C., Park Y. S., Kim E. H., Oligonucleotide chip
composition for analyzing hepatitis c virus (hcv)
genotype and detecting method thereof, 2004, Patent
US2004170957.
Pass R. F., Cytomegalovirus infection, 2002 Pediatr. Rev.
Vol.23, pp. 163–170.
Pultoo A., Jankee H., Meetoo G., Pyndiah M. N., Khittoo
G., Detection of cytomegalovirus in urine of hearing-
impaired and mentally retarded children by PCR and
cell culture, 2000, J. Commun. Dis. Vol. 32, pp. 101–
108.
Raynor B. D., Cytomegalovirus infection in pregnancy,
1993, Semin. Perinatol. Vol.17, pp. 394-402.
Revello M. G., Gerna G., Diagnosis and management of
human cytomegalovirus infection in the mother, fetus,
and newborn infant, 2002, Clin. Microbiol. Rev. Vol.
15, pp. 680–715.
Stagno S., Pass R. F., Cloud G., Britt W. J., Henderson R.
E., Walton P. D., Veren D. A., Page F., Alford C. A.,
Primary cytomegalovirus infection in pregnancy.
Incidence, transmission to fetus, and clinical outcome,
1986, JAMA Vol. 256, pp. 1904–1908.
Yinon Y., Farine D., Yudin M. H., Gagnon R., Hudon L.,
Basso M., Bos H., Delisle M. F., Menticoglou S.,
Mundle W., Ouellet A., Pressey T., Roggensack A.,
Boucher M., Castillo E., Gruslin A., Money D. M.,
Murphy K., Ogilvie G., Paquet C., Van Eyk N., Van
Schalkwyk J., Cytomegalovirus infection in
pregnancy, 2010, J. Obstet. Gynaecol. Can. Vol. 32,
pp. 348-354.
BIODEVICES 2011 - International Conference on Biomedical Electronics and Devices
108