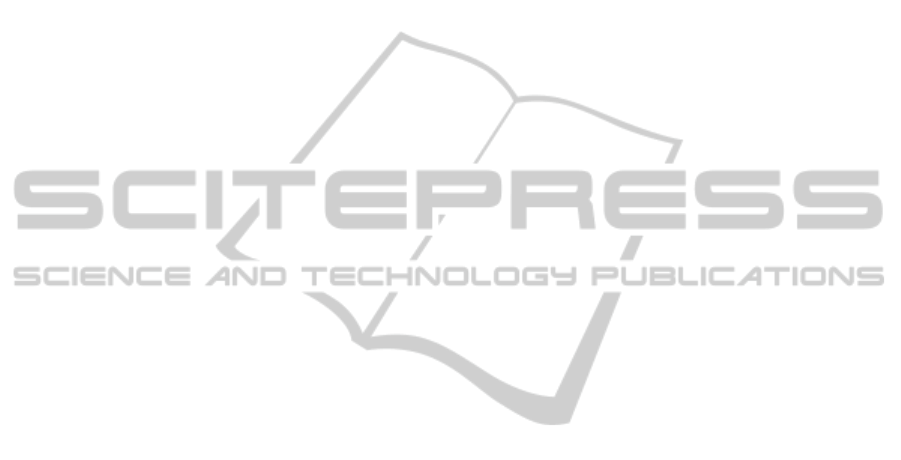
Faniran, J. and Shurvell, H. (1968). Infrared spectra
of phenylboronic acid (normal and deuterated) and
diphenyl phenylboronate. Canadian Journal of Chem-
istry, 46(12):2089–2095.
Fl¨uckiger, R., Woodtli, T., and Berger, W. (1984). Quantita-
tion of glycosylated hemoglobin by boronate affinity
chromatography. Diabetes, 33(1):73.
Frantzen, F., Grimsrud, K., Heggli, D., Faaren, A., Lovli, T.,
and Sundrehagen, E. (1997). Glycohemoglobin filter
assay for doctors’ offices based on boronic acid affin-
ity principle. Clinical chemistry, 43(12):2390.
Geim, A. and MacDonald, A. (2007). Graphene: Exploring
carbon flatland. Physics Today, 60:35.
Geim, A. and Novoselov, K. (2007). The rise of graphene.
Nature materials, 6(3):183–191.
Halwachs-Baumann, G., Katzensteiner, S., Schnedl, W.,
Purstner, P., Pieber, T., and Wilders-Truschnig, M.
(1997). Comparative evaluation of three assay sys-
tems for automated determination of hemoglobin A1c.
Clinical chemistry, 43(3):511.
Jeppsson, J., Kobold, U., Barr, J., Finke, A., Hoelzel,
W., Hoshino, T., Miedema, K., Mosca, A., Mauri,
P., Paroni, R., et al. (2002). Approved IFCC refer-
ence method for the measurement of HbA1c in human
blood. Clinical Chemistry and Laboratory Medicine,
40(1):78–89.
Liu, S., Wollenberger, U., Katterle, M., and Scheller, F.
(2006). Ferroceneboronic acid-based amperometric
biosensor for glycated hemoglobin. Sensors and Ac-
tuators B: Chemical, 113(2):623–629.
Menard, L., Dempsey, M., Blankstein, L., Aleyassine, H.,
Wacks, M., and Soeldner, J. (1980). Quantitiative de-
termination of glycosylated hemoglobin A1 by agar
gel electrophoresis. Clinical Chemistry, 26(11):1598.
Park, S., Dikin, D., Nguyen, S., and Ruoff, R. (2009).
Graphene Oxide Sheets Chemically Cross-Linked by
Polyallylamine. The Journal of Physical Chemistry C,
113(36):15801–15804.
Peterson, K., Pavlovich, J., Goldstein, D., Little, R., Eng-
land, J., and Peterson, C. (1998). What is hemoglobin
A1c? An analysis of glycated hemoglobins by elec-
trospray ionization mass spectrometry. Clinical chem-
istry, 44(9):1951.
Privett, B., Shin, J., and Schoenfisch, M. (2008).
Electrochemical sensors. Analytical chemistry,
80(12):4499–4517.
Ramesh, P., Bhagyalakshmi, S., and Sampath, S. (2004).
Preparation and physicochemical and electrochemical
characterization of exfoliated graphite oxide. Journal
of colloid and interface science, 274(1):95–102.
Rohovec, J., Maschmeyer, T., Aime, S., and Peters, J.
(2003). The structure of the sugar residue in glycated
human serum albumin and its molecular recognition
by phenylboronate. Chemistry–A European Journal,
9(10):2193–2199.
Schedin, F., Geim, A., Morozov, S., Hill, E., Blake, P., Kat-
snelson, M., and Novoselov, K. (2007). Detection of
individual gas molecules adsorbed on graphene. Na-
ture Materials, 6(9):652–655.
Silverstein, R. and Bassler, G. (1962). Spectrometric iden-
tification of organic compounds. Journal of Chemical
Education, 39(11):546.
Son, S. and Yoon, H. (2008). Electrochemical analysis of
glycated hemoglobin based on the biospecificity and
electron-transferring capability of ferroceneboronic
acid. BioChip J, 2:116–122.
Springsteen, G. and Wang, B. (2002). A detailed examina-
tion of boronic acid-diol complexation. Tetrahedron,
58(26):5291–5300.
Sun, X., Liu, Z., Welsher, K., Robinson, J., Goodwin, A.,
Zaric, S., and Dai, H. (2008). Nano-graphene oxide
for cellular imaging and drug delivery. Nano research,
1(3):203–212.
Takahashi, S. and Anzai, J. (2005). Phenylboronic acid
monolayer-modified electrodes sensitive to sugars.
Langmuir, 21(11):5102–5107.
Zhao, Y., Luo, H., and Li, N. (2009). Electrochemical
characterization of in situ functionalized gold p-
aminothiophenol self-assembled monolayer with 4-
formylphenylboronic acid for recognition of sugars.
Sensors and Actuators B: Chemical, 137(2):722–726.
Zuo, X., He, S., Li, D., Peng, C., Huang, Q., Song, S.,
and Fan, C. (2009). Graphene Oxide-Facilitated Elec-
tron Transfer of Metalloproteins at Electrode Sur-
faces. Langmuir, 26(3):1936–1939.
DETECTION OF GLYCATED HEMOGLOBIN USING 3-AMINOPHENYLBORONIC ACID MODIFIED GRAPHENE
OXIDE
113