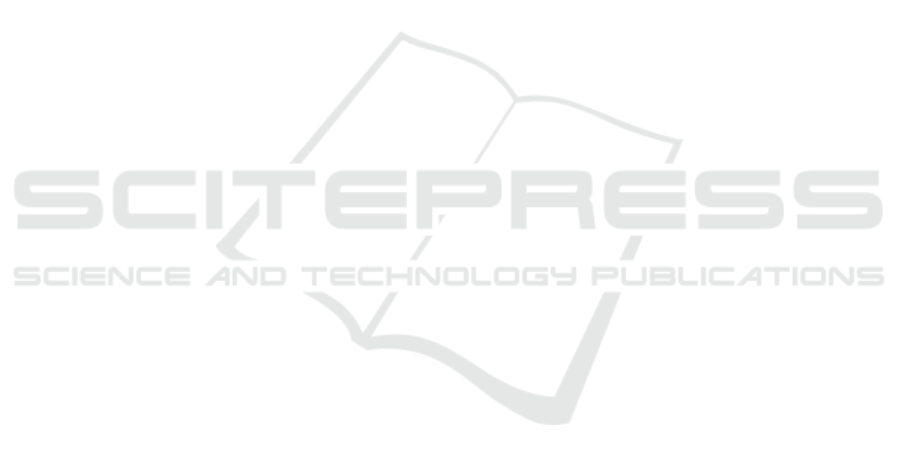
MRI-INDUCED SAR ON PACEMAKER LEADS
Numerical Simulations on Three Human Phantoms
Eugenio Mattei, Giovanni Calcagnini, Michele Triventi, Federica Censi and Pietro Bartolini
Italian National Institute of Health, Department of Technology and Health, Rome, Italy
Keywords: Magnetic Resonance Imaging, Pacemaker, Specific Absorption Rate, Human Visible Dataset.
Abstract: Numerical simulations were performed to evaluate the Specific Absorption Rate (SAR) induced at the tip of
a pacemaker (PM) implant by the 64 MHz radiofrequency (RF) field used in 1.5T Magnetic Resonance
Imaging (MRI) procedures. The analysis was performed by using a commercial FDTD software (SEMCAD
X, SPEAG, Switzerland) and aimed at the evaluation of the impact that the patient ‘s morphology has on the
induced local SAR at the implant tip. In particular three human phantoms were studied: a 34-year old man
model, a 26-year old woman, and a 6-year old boy. The three phantoms reproduce more than 70 tissues of
the human body with a resolution of 1 mm. Inside each phantoms, realistic implant configurations were
modelled, considering both left and right pectoral implants, and atrial and ventricular stimulations. The local
SAR values at the lead tip was compared for the three phantoms and sensible differences were observed:
with a RF excitation set to produce an whole-body average SAR of 2 W/kg without any implants, local SAR
values ranged from 641W/kg (woman model – right ventricular implant) to 3 W/kg (boy model – left atrium
implant). We also observed that, in general, ventricular implants showed a higher SAR compared to atrial
ones, as well as right pectoral implants compared to left ones. However, not always a higher implant area or
a longer lead path implied higher SAR at the tip, indicating the coupling mechanisms between the implant
and the RF field are likely to be more complex that the only area-dependent induction law.
1 INTRODUCTION
The number of Magnetic Resonance Imaging (MRI)
scans performed annually has increased dramatically
over the past few years. Parallel to the growth and
evolution of the MRI field, is the burgeoning
number of patients benefiting from implantable
cardiac systems including pacemaker (PM) and
implantable cardioverter/defibrillators (ICDs). The
combination of these two growing phenomena
results in an estimated 50-75% probability of a
patient being indicated for an MRI study over the
lifetime of their device; it has created an estimated
200,000 implanted patients who were denied the
MRI scan, and this numbers are likely to increase in
the future (Rougin et al, 2004). Given the rapid
expansion of technology in the fields of both MRI
and device arrhythmia management, there is
increasing interest in the issue of implantable device
safety in the MRI environment. For the purpose of
MRI, non-ferromagnetic material is available for
manufacturing of implantable devices. Considering
the impressive progress in the use of diamagnetic
material, the most important safety problem
associated with MRI and medical implantable
devices is the potential tissue heating induced by the
radiofrequency (RF) fields. In this filed, numerical
studies are crucial to extend the range of
experimental measurements and to correlate heating
results to those expected in humans. Simulations can
be used to model realistic patient geometries, to
deliver more information (e.g., 3D fields instead of
single measurement points) and to study individually
the impact of parameters such as tissue properties,
boundary conditions, etc. As the finite difference
time domain (FDTD) method has been a widely
used technique for characterization of RF heating, it
is an excellent candidate to render an accurate
estimate of the specific absorption rate (SAR), and
therefore heating, due to implantable devices during
MRI experiments.
The anatomical structure of the human body as
sets of minute elements (voxels) suitable to be
imported inside the FDTD environment have been
obtained from MRI scans, X-ray computed
tomography, or anatomical coloured images of the
135
Mattei E., Calcagnini G., Triventi M., censi F. and Bartolini P..
MRI-INDUCED SAR ON PACEMAKER LEADS - Numerical Simulations on Three Human Phantoms.
DOI: 10.5220/0003131501350139
In Proceedings of the International Conference on Biomedical Electronics and Devices (BIODEVICES-2011), pages 135-139
ISBN: 978-989-8425-37-9
Copyright
c
2011 SCITEPRESS (Science and Technology Publications, Lda.)