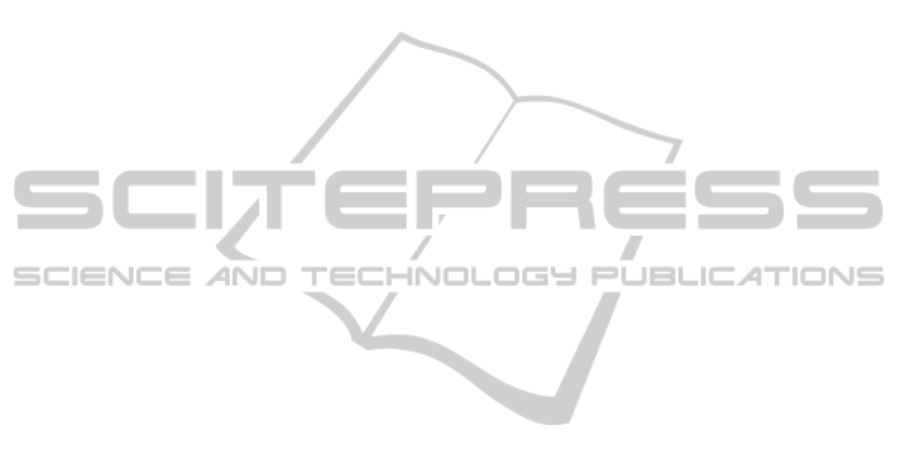
identical, in keeping with previous findings
(Reinvuo, 2006) and (Hung, 2008). However, this
study is the first to show that voluntary changes in
the depth of breathing can also be accurately
detected by the device.
This study is also the first to demonstrate that the
motor pattern of voluntary cough can successfully be
distinguished from that of normal breathing by
means of an accelerometer pair. In addition, the
accelerometric pattern of cough was fairly coherent
with that recorded spirometrically, and revealed high
frequency components due to speed of the expulsive
activity and related chest wall vibrations. However,
inaccuracies still exist as far as the accelerometric
evaluation of cough intensity is concerned.
Accelerometric long-term recordings of
cardiorespiratory activity during sleep in normal
subjects provide some advantages compared with
standard techniques. Although not formally tested
here, it seems obvious that our device offers better
portability than the conventional ones and that the
possibility of simultaneous recording of body
posture may represent an important tool in the
evaluation of sleep disturbances.
It could be argued that, in order to monitor
cardiorespiratory activity, the use of an
accelerometer-based device is somewhat limited by
the interfering signals of body motion, especially
during daily activities. Whilst we acknowledge that
this limitation may exist at least to some extent, we
also feel that the recording a cardiorespiratory signal
“disturbed” by that originating from body
movements is “per se” of clinical usefulness. It may
be inferred that patients with respiratory
disturbances must present a ratio of resting-to-
activity time that is inversely related to the general
clinical condition. Therefore, an increase in the
above time ratio could be interpreted as an index of
a deteriorating clinical condition. In addition, in
patients with respiratory diseases, the detection of an
increase in cardiac and respiratory activity at rest
could also point to an increased metabolic demand
such as in the event of a respiratory exacerbation.
5 CONCLUSIONS
Simultaneous recordings of respiratory movements,
heart rate and body position can easily be
accomplished by using pairs of tri-axial
accelerometers; these devices seem to be also
suitable for the detection of the motor pattern of
cough.
The device can be employed for daytime and
nocturnal long-term monitoring thanks to its small
dimensions, small weight and easy positioning of
sensors on the chest wall, that warrant non-invasive
measurements. It could be employed in the diagnosis
of sleep disturbances such as the sleep apnoea
syndrome, or in the monitoring of the elderly. Even
at the present stage of development, the device
presented here appears to be ready for accurate and
reliable long-term sleep studies. Being easily
portable and not bulky, the device seems to be
particularly suitable for sleep studies in the domestic
environment.
REFERENCES
Tobin, M. J., Chadha, T. S., Jenouri, G., Birch, S. J.,
Gazeroglu, H. B., Sackner, M. A., 1983. Breathing
patterns. 1. Normal subjects. In Chest; 84 (2): 202-5.
Pauwels, R. A., Buist, A. S., Calverley, P. M., Jenkins, C.
R., Hurd, S. S., 2001. Global strategy for the
diagnosis, management, and prevention of chronic
obstructive pulmonary disease. NHLBI/WHO Global
Initiative for Chronic Obstructive Lung Disease
(GOLD) Workshop summary. In Am J Respir Crit
Care Med; 163(5):1256-76.
Britton, M., 2003. The burden of COPD in the U.K.:
results from the Confronting COPD survey. In Respir
Med; 97 Suppl C:S71-9.
Panossian, L. A., Avidan, A. Y., 2009. Review of sleep
disorders. In Med Clin North Am.; 93(2):407-25.
Redline, S., Young, T., 1993. Epidemiology and natural
history of obstructive sleep apnea. In Ear Nose Throat
J; 72(1):20-1, 24-6.
Deegan, P. C., McNicholas, W. T., 1995. Pathophysiology
of obstructive sleep apnoea. In Eur Respir
J;8(7):1161-78.
McNicholas, W. T., 2008. Diagnosis of obstructive sleep
apnea in adults. In Proc Am Thorac Soc;5(2):154-60.
Hurst, J. R., Wedzicha, J. A., 2009. Management and pre-
vention of chronic obstructive pulmonary disease
exacerbations: a state of the art review. In BMC Med;7:40.
Perez, W., Tobin, M. J., 1985. Separation of factors
responsible for change in breathing pattern induced by
instrumentation. In J Appl Physiol; 59 (5):1515-20.
Que, C. L., Kolmaga, C., Durand, L. G., Kelly, S. M.,
Macklem, P. T., 2002. Phonospirometry for non-
invasive measurement of ventilation: methodology and
preliminary results. In J Appl Physiol;93(4):1515-26.
Reinvuo, T., Hannula, M., Sorvoja, H., Alasaarela, E.,
Myllyla, R., 2006. Measurement of respiratory rate
with high-resolution accelerometer and emfit pressure
sensor. In Sensors Applications Symposium, 2006.
Proceedings of the 2006 IEEE , vol., no., pp. 192- 195,
Hung, P. D., Bonnet, S., Guillemaud, R., Castelli, E., Yen,
P. T. N., 2008. Estimation of respiratory waveform
using an accelerometer. In Biomedical Imaging: From
Nano to Macro, 2008. ISBI 2008. 5th IEEE
International Symposium on, vol., no., pp.1493-1496,
14-17 May
BIOSIGNALS 2011 - International Conference on Bio-inspired Systems and Signal Processing
42