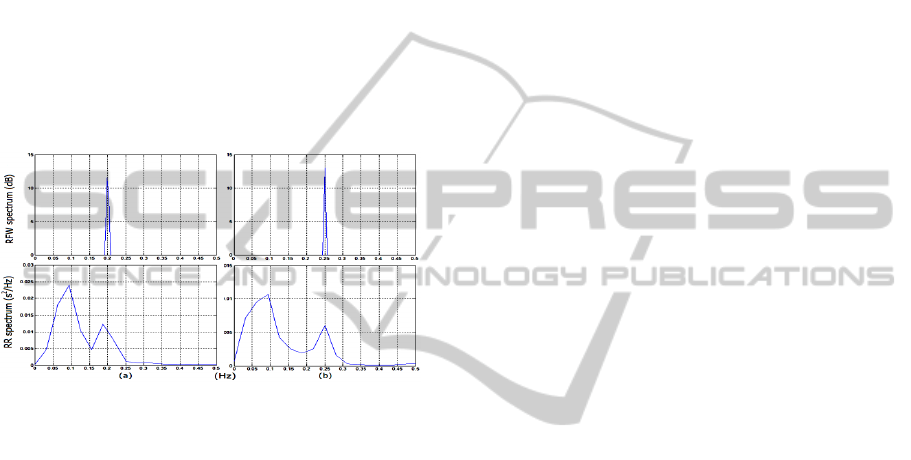
signals can be registered without any cable
connection, reducing sources of artifacts and
providing mobility during the recordings. The
conditioning circuits demonstrated suitable gains for
the desired measurements and good immunity
against spurious interferences during recordings in
the Hospital. Two ECG leads are acquired by the
device to avoid data loss. In case of electrode
displacement or deterioration of the electrode-skin
interface, the RR interval can be still calculated
using the other lead. Concerning the transmission
distance, the Bluetooth connection proved to be
reliable (no data loss) over a range of 20 m even
with obstacles. The elimination of the cables besides
reducing artifacts, provides more comfort to the
subjects during the recordings.
Figure 6: Example of respiratory modulation of the HRV.
The breathing rate was increased from 0.2 Hz (a) to 0.25
Hz (b) as can be seen in the RFW spectrum. As result, the
HF peak of the HRV is also shifted in the RR spectrum.
The PPG signal provides information on the
long-term changes of the mean ABP that can be used
together with the PPG-AC to better estimate the
systolic and diastolic pressure in future researches.
The presented results are similar to the ones
obtained by Chen and Mukkamala (2008) who used
propranolol, a non-selective β blocker. Signals of
other volunteers are being acquired as described
above to set a data bank that will be used to
investigate indexes that may better characterize the
ANS activity.
5 CONCLUSIONS
A wireless non-invasive system for the recording of
cardio-respiratory signals was described. Due to its
portability, the system can be used in different
scenarios, being a valuable tool for research.
The synchronized recordings of ECG, RFW and
PPG allow a better assessment of the interactions
among cardio-respiratory variables and their effect
on the HRV.
Digital signal processing techniques and
mathematical modelling will be applied to the
acquired data to further investigate the
cardiovascular regulation by the ANS.
REFERENCES
Akselrod, S., Gordon, D., Madwed, L. B., Snidman, N. C.,
Shannon, D. C., Cohen, R. J., 1985. “Hemodynamic
regulation: investigation by spectral analysis”. Am J
Physiol Heart Circ Physiol, 249: H867–H875.
Berger, R. D.; Saul, J. P.; Cohen, R. J., 1989. “Assessment
of Autonomic Response by Broad-Band Respiration.
IEEE TRANSACTIONS ON BIOMEDICAL
ENGINEERING, vol. 36, n. II.
Brown, T. E., Beightol, L. A., Koh, J., Eckberg, D. L.,
1993. “Important influence of respiration on human R-
R interval power spectra is largely ignored”. J Appl
Physiol. Nov;75(5):2310-7.
Cavalcanti, S., 2000. “Arterial baroreflex influence on
heart rate variability: a mathematical model-based
analysis”. Med. Biol. Eng. Comput., 38, 189-197.
Chen, X., Mukkamala, R., 2008. “Selective quantification
of the cardiac sympathetic and parasympathetic
nervous systems by multisignal analysis of
cardiorespiratory variability”. Am J Physiol Heart Circ
Physiol. 294: H362-H371.
Ghaffari, A., Golbayani, H., Ghasemi, M., 2008. “A new
mathematical based QRS detector using continuous
wavelet transform”. Computers and Electrical
Engineering, v. 34, p. 81-91.
Jose, A. D., Taylor, R. R. 1969. “Autonomic blockade by
propranolol and atropine to study intrinsic myocardial
function in man”. The Journal of Clinical
Investigation. November; 48(11): 2019–2031.
Lass, J., Meigas, K., Karai, D., Kattai, R., Kaik, J.,
Rossmann, M. 2004. “Continuous blood pressure
monitoring during exercise using pulse wave transit
time measurement”. Proc. IEEE EMBS. Sept 1-5.
Parati, G., Saul, J. P., Di Rienzo, M., Mancia, G., 1995.
“Spectral analysis of blood pressure and heart rate
variability in evaluating cardiovascular regulation – A
critical appraisal”. Hypertension. 25:1276-1286.
Parati, G., Mancia, G., Rienzo, M. D., Castiglioni, P.,
Taylor, J. A., Studinger, P., 2006.
“Point:Counterpoint: Cardiovascular variability is/is
not an index of autonomic control of circulation”. J Ap
Phy,: 676–682.
TASK FORCE OF THE EUROPEAN SOCIETY OF
CARDIOLOGY AND THE NORTH AMERICAN
SOCIETY OF PACING AND
ELECTROPHYSIOLOGY, 1996. “Heart rate
variability: standards of measurement, physiological
interpretation, and clinical use.” Circ., 93:1043–1065.
WIRELESS DEVICE FOR NONINVASIVE RECORDINGS OF CARDIO-RESPIRATORY SIGNALS
367