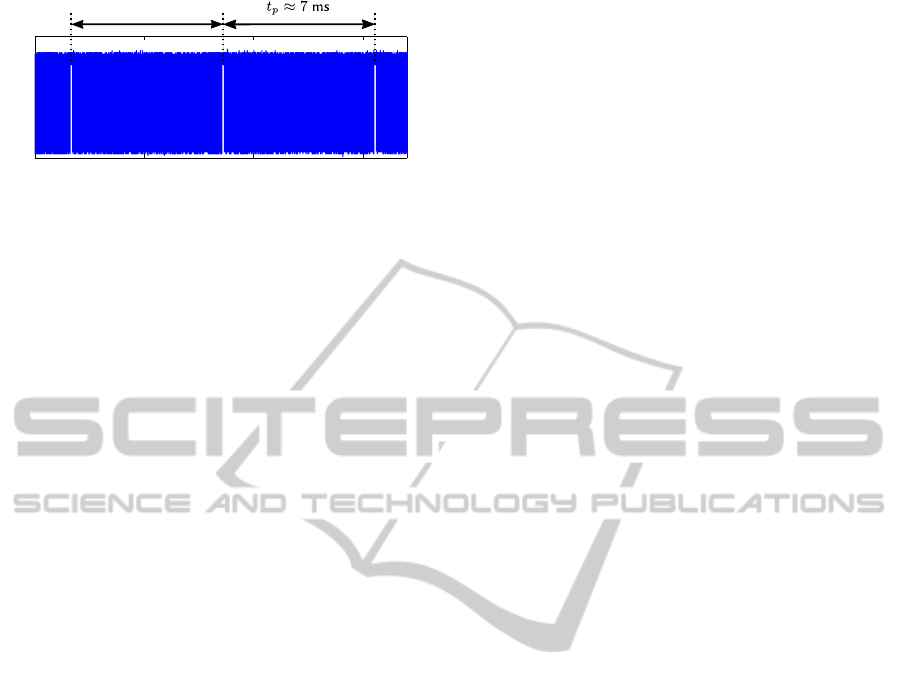
0 . 0 0 5 0 . 0 1 0 . 0 1 5 0 . 0 2
0
2
4
Time [s]
V
e x
[V]
Figure 4: The 32 kHz excitation signal shows gaps every
7 ms, resulting in 140 Hz common-mode noise in the ECG
signal if no further measures are taken.
Final Artifact Marker Signal. Supplementary to
the artifact indicator, we have taken an additional pa-
rameter into account: using the original ECG data, we
verify that the signal lies within the range of [10, 90]%
LSB
ADC
of the analog-digital-converter, in order to
eliminate regions where the signal is near saturation.
Then the artifact indicator and the in-range indica-
tor were logically ANDed to generate the final artifact
marker signal. When this signal is high, the corre-
sponding region in the ECG signal is marked as arti-
fact region. Furthermore, artifact regions that do not
have a minimum distance of 1 s are joined.
6 EVALUATION & RESULTS
With the system presented above, we have conducted
measurements with several subjects. The driven-
seat circuit was not necessary because the systems’
coupling to earth ground was very weak due to the
battery-powered, wireless concept. Yet with the ca-
pacitance measurement circuit enabled, we observed
a large amount of common-mode noise on the ECG
signal with a frequency of approximately 140 Hz, the
source of which was not obvious at first. A closer
look at the excitation signal of the AD7152 revealed
that even though the device was used in one-channel
differential mode with continuous conversion, gaps in
the excitation signal occurred with a distance of ap-
proximately 7 ms (see Figure 4). This was visible as
common-mode noise in the ECG signal.
Enabling the driven-seat circuit to remove this
noise was not an option, as a 180
◦
phase shift is neces-
sary to destructively interfere with the undesired fre-
quencies. In the case of 140 Hz, this would result in a
rather high bandwidth of the driven-seat stage. Then,
the excitation signal of 32 kHz would have been at-
tenuated too, resulting in incorrect capacitance mea-
surement values. The solution for us was to simply
narrow the bandwidth of the CCEs by adding 50 Hz
low-pass filters.
6.1 Artifact Signal
The proposed algorithm generates an artifact signal
with very high dynamics. For slight capacitance
changes generated by breathing and light movements,
the signal amplitudes stay very low. Higher move-
ment amplitudes can increase the artifact amplitudes
up to 4 orders of magnitude. Hence, a threshold could
be identified that separates regular ECG episodes
from the ones with artifacts. First results showed, that
no adaptation of the threshold was necessary for dif-
ferent subjects.
6.2 Sample Measurement
Figure 5 finally shows an example of the computa-
tion of artifact regions for a capacitive ECG signal
recorded with the system proposed in this paper. The
gray trace represents the artifact level generated by
adaptive filtering of the differential capacitance values
captured by the AD7152. Above a certain threshold,
the artifact indicator goes high. The artifact region
can be marked in the ECG signal.
7 SUMMARY & OUTLOOK
Non-contact capacitive ECG systems represent an un-
obtrusive way to acquire cardiovascular data. Yet, due
to the measurement principle, these systems are sensi-
tive to motion artifacts. For automatic signal analysis,
a means of estimating signal quality is therefore de-
sired.
Combining a capacitive ECG measurement sys-
tem with a capacitance-to-digital conversion de-
vice, we have presented an unobtrusive ECG sys-
tem that continuously monitors the electrode-body-
capacitance. This capacitance value represents a con-
text signal that can be used to derive a quality signal
for the capacitive ECG measurement.
Using adaptive filtering and post-processing we
were able to show first results of the system perfor-
mance. With the system, it is possible to mark re-
gions with artifacts in the capacitive ECG signal, and
exclude them from further processing thus improving
automatic analyzability.
Currently we are building a database with mea-
surements recorded with our system. With the data
we will be able to quantify the improvement of au-
tomatic analysis of unobtrusively acquired ECG data
due to our method. For automatic QRS detection, a
considerable decrease in false (positive and negative)
detections due to the proposed method should be pos-
sible, yielding an increase in sensitivity and positive
SIGNAL QUALITY ASSESSMENT FOR CAPACITIVE ECG MONITORING SYSTEMS USING
BODY-SENSOR-IMPEDANCE
457