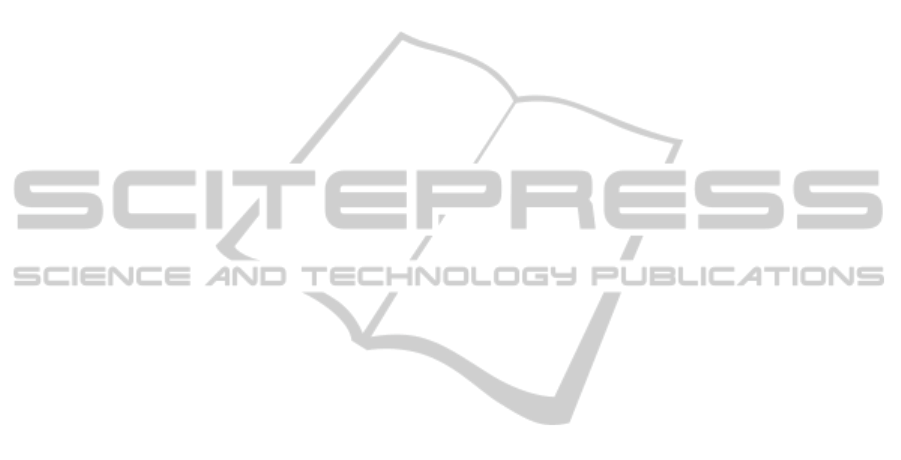
implant within field regulations is the ultimate goal
of this work. In order to determine this, five different
coils were wound with different winding-core ratios
and their behaviour was investigated at different
frequencies. The performance of the system was
determined through circuit design, analysis and
experimentation. Practically, the transmitter coil was
supplied with current from a function generator,
where current levels were limited so that their
associated magnetic fields were lower than specified
by regulatory bodies. The receiver coil was placed at
a distance (55 mm) from the transmitter coil, and
voltage levels were measured under different
operating frequencies.
The results of this work indicate that an optimum
coil-core ratio for a certain receiver coil size is one
in which the cross sectional area of the winding is
approximately equal to that of the core. Average
power levels of up to 4.3 μW are demonstrated
within electromagnetic field regulations for a 5 mm
diameter / 10 mm long receiver coil when located 55
mm from a transmitter coil. Pulsed power levels of
up to 12 mW are illustrated.
Conditions for maximum power transfer are
analyzed on the basis of matching the load with the
receiver circuit impedance. It was found that there is
significant variation in coil resistance with
frequency and that this impacts on the maximum
power that can be transmitted. The power transfer
capability of each coil is illustrated in terms of the
maximum power it can transmit to matched loads at
different frequencies. Work is ongoing to customise
the receiver and transmitter coil designs for specified
load impedance values, which are typical of those
encountered in biomedical applications. The impact
of different core materials and wire dimensions will
also be investigated both for the transmitter and
receiver coils.
ACKNOWLEDGEMENTS
This work is supported by Enterprise Ireland and
Brivant Ltd. under the Innovation Partnership
Programme, Grant no. IP/2007/0447.
REFERENCES
Ahmadian, M., B. W. Flynn, et al. (2005). "Data
transmission for implantable microsystems using
magnetic coupling." communications, IEEE
proceedings- 152(2): 247-250.
Ali, H., T. J. Ahmad, et al. (2009). Inductive link design
for medical implants. Industrial electronics &
applications, 2009. ISIEA 2009. IEEE symposium.
Atluri, S. And M. Ghovanloo (2005). Design of a
wideband power-efficient inductive wireless link for
implantable biomedical devices using multiple
carriers. Neural engineering, 2005. Conference
proceedings. 2nd international IEEE EMBS
conference.
Clements, M., K. Vichienchom, et al. (1999). An
implantable power and data receiver and neuro-
stimulus chip for a retinal prosthesis system. Circuits
and systems, 1999. ISCAS '99. Proceedings of the
1999 IEEE international symposium.
Djordje Popovic, l. L. B., and Gerald E. Loeb, senior
member, IEEE (2007). "recruitment and comfort of
Bion implanted electrical stimulation: implications for
FES applications." IEEE transactions on neural
systems and rehabilitation engineering 15(4).
Fair-rite. "fair-rite products corp. PO box J,One
commercial row, Wallkill, NY 12589-0288", 2010,
from www.fair-rite.com.
Flynn, K. F. A. B. W. Wireless powering of implanted
sensors using RF inductive coupling.
Fotopoulou, K. And B. W. Flynn (2006). Wireless
powering of implanted sensors using RF inductive
coupling. Sensors, 2006. 5th IEEE conference.
Furse, C. M., R. Harrison, et al. (2007). Recent advances
in biomedical telemetry. Electromagnetics in advanced
applications, 2007. ICEAA 2007. International
conference.
Harrison, R., P. Watkins, et al. (2006). A low-power
integrated circuit for a wireless 100-electrode neural
recording system. Solid-state circuits conference,
2006. ISSCC 2006. Digest of technical papers. IEEE
international.
Hmida, G. B., M. Dhieb, et al. (2006). Transcutaneous
power and high data rate transmission for biomedical
implants. Design and test of integrated systems in
nanoscale technology, 2006. DTIS 2006. International
conference.
IEEE standard. (1999). "IEEE standard for safety levels
with respect to human exposure to radio frequency
electromagnetic fields, 3 kHz to 300 GHz, 1999."
J. H. Schulman, J. P. M., J. Wolfe, E. Regev, C. Y. Perron,
R. Ananth, E. Matei, A. Glukhovsky, R. Davis (2004).
Battery powered Bion FES network. 26th annual
international conference of the IEEE EMBS San
Francisco, CA, USA.
Kihyun Jung, Y.-H. K., Euy Jung Choi, Hyun Jun Kim,
and Yong-Jun Kim (2008). Wireless power
transmission for implantable devices using inductive
component of closed-magnetic circuit structure. IEEE
international conference on multisensor fusion and
integration for intelligent systems. Seoul, Korea.
Maxwell2d. "ansoft maxwell 2d." from www.ansoft.com.
Mounaim, F., M. Sawan, et al. (2009). Integrated
inductive power and data recovery front-end dedicated
to implantable devices. Biomedical circuits and
systems conference, 2009. BIOCAS 2009. IEEE.
OPTIMUM CORE-WINDING RATIO FOR A RECEIVER COIL IN A BIOMEDICAL INDUCTIVE POWER SYSTEM
85