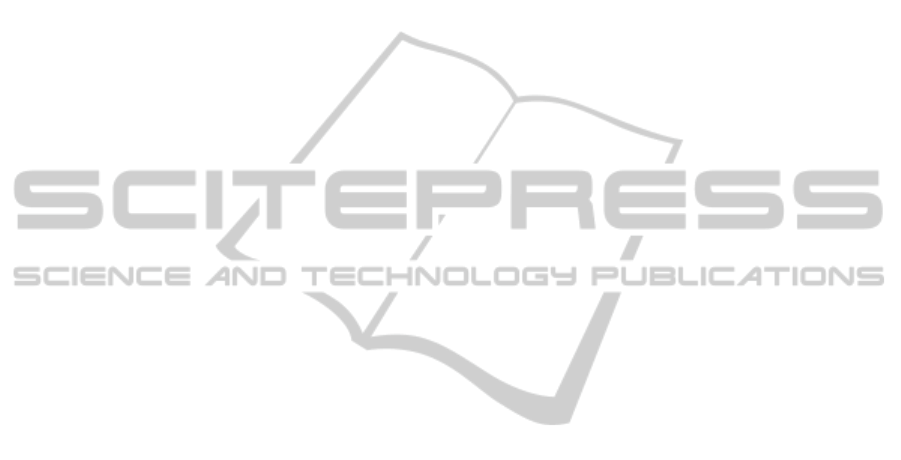
structural layers. MEMS can act as sensors or
actuators, either individually or in arrays, to produce
effects on a larger scale.
Currently, there is a large number of different
MEMS. Non-comprehensive examples are pressure
and displacement sensors, accelerometers,
gyroscopes, cantilevers, precision instruments,
manipulators, micro-relays, micromirrors, thermal,
chemical, micro-fluidics, etc. Each MEMS device
requires its own electronic circuitry, based on
characteristic frequency, load, voltage and current
levels, noise, etc. For instance, for a capacitive
accelerometer the resonance frequency ranges in the
order of tens of kHz, bandwidth of several kHz,
under a 6 V supply. For an angular quartz sensor, the
vibration is of some kHz and bandwidth over 50 Hz,
with voltage over 5 V. Other kinds of MEMS
require much higher frequency, such as those
operating in RF. In electrostatic actuators, voltage
requirements may be tens of volts or more.
Therefore, treatment should be completely different
for each particular case.
Besides the previous consideration, MEMS
sensors and actuators characteristics variation with
aging, temperature, humidity or other physical
phenomena, as well as reliability, raise the need for a
self-adaptive conditioning systems.
Therefore, MEMS/NEMS already offer and
promise more interaction with the environment at
micro/nano scale. Thus, embedded in computing
nodes, reduced-size and low-power systems able to
interact with the environment become feasible.
MEMS technologies are not CMOS compatible
in general, so further integration is limited from this
point of view. Several research efforts in developing
compatible CMOS MEMS have been done in the
latest years, as it will be discussed (Baltes H. et al.,
2002; Brand O. et al., 2005).
1.3 Self-adaptation and Bioinspiration
Despite technology already offers powerful
computing and sensing devices, artificial algorithms
still are limited in the extent of environment
interaction capabilities, compared with even the
simpler existing living beings.
The inspiration more directly related to
environment perception is the autonomous function
of the human central nervous system. The human
autonomous control employs motor neurons to send
indirect messages to organs at a subconscious level.
These messages regulate unconscious processes and
variables such as temperature, breath, heartbeat or
digestion, among many others. The parallel for
artificial systems is a network of processors which
performs the necessary operations at the right time
without the need of dedicated attention, in the so-
called autonomic computing paradigm (Kephart, J.
O.; Chess, D. M., 2003).
Such computing paradigm changes from the
conventional processing power to another one driven
by data. Besides the traditional centralized storage,
access to data from multiple distributed sources
enable users to access to information when and
where needed. The main objectives of these
distributed and autonomous architectures are often
referred to as self-* properties. Systems able to self-
manage should be self-configuring, self-healing,
self-optimizing, and self-protecting and exhibit self-
awareness, self-situation, self-monitoring, and self-
adjustment (Dobson, S. et al., 2010). Thus, the
information they can provide from the physical
environment they are immersed is essential for those
systems.
In addition to the autonomic computation
proposal, there are many other similar approaches,
for example Organic Computing (Gudemann, M. et
al. 2008) Systemic Computation (Bentley, P. J.,
2007) and Æther (Soto, V. J. et al., 2009).
Due to the power and interconnect limitations,
increased processor performance is nowadays
coming more from the increasing computing
parallelism rather than from clock frequency
improvements. The nanometer-size cutting-edge
VLSI devices suffer from great variability and non-
ideal effects. Further, with reduced dimensions,
cosmic radiation-produced soft errors start to appear
also at ground level. For these reasons, very serious
reliability issues arise and a holistic strategy for fault
tolerance and self-repair is required.
Because of their nature, bio-inspired neural
networks promise feasible solutions: they are
constructed with slow and unreliable elements, they
are tolerant to manufacturing defects and to noisy
environments, they are robust in the presence of
hardware failures, they are not programmed but they
adapt and self-organize, and they interact with the
real world.
Based on studies of the human cortex the fields
of computer science and cognitive neuroscience
have been combined from a top-down approach
(Hawkins J. and Blakeslee S., 2004). It is
conjectured that the resolution of complex
perception problems is done by means of few layers
of neurons, evenly distributed and massively parallel
working further massively interconnected with direct
and feedback flows of information. The ability to
predict is what sets the human intelligence and it is
BIOINSPIRED SENSORY INTEGRATION FOR ENVIRONMENT-PERCEPTION EMBEDDED SYSTEMS
261