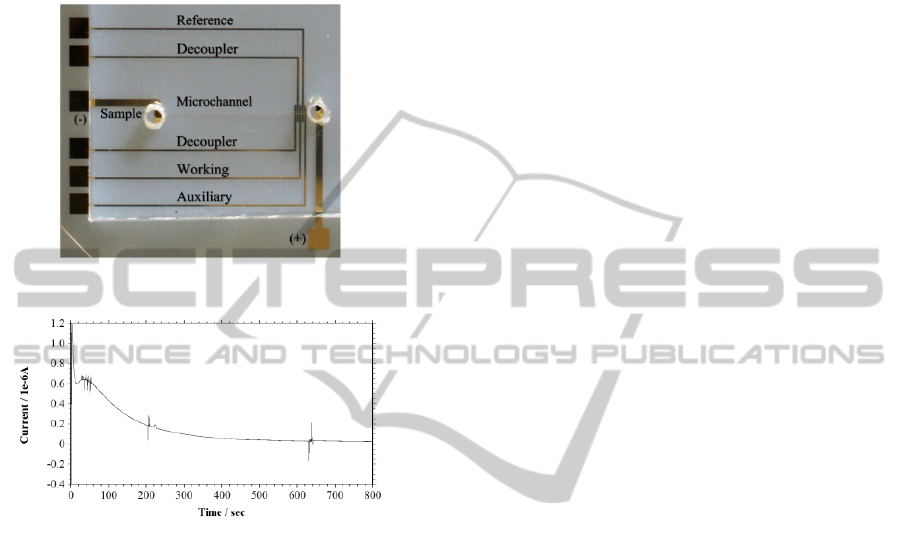
the i-t curve of MB-DNA detection through CE-AD.
Different peaks resemble MB attached to various
bands of the DNA. The peak was detected after 2
min of sample injection. The detection sensitivity
and LOD for this reaction were 640 nA/μg of DNA
and 140 ng of DNA respectively.
Figure 5: CE-AD microchip.
Figure 6: i-t curve of MB-DNA complex in KCl 200 mM.
Therefore, it would be possible to first determine
peak current for MB from CE-AD experiment and
then decipher its concentration using standard curve
obtained under control conditions (Figure 4).
Thereafter, DNA concentration can be calculated
from the standard value obtained. This shows the
potential of present study towards successful
detection of DNA of any sizes and deciphers its
concentration on our microchip. Further studies are
underway to enhance the reproducibility and
sensitivity of these devices and for detection of a
mixture of short DNA fragments such as found in
DNA molecular weight markers.
4 CONCLUSIONS
It was concluded in the present study that disposable
CE-AD microchips with gold electrodes and PDMS
channels can be used to prepare effective DNA
detector in place of existing gel electrophoresis
based system. The disposable electrochemical
detector fabricated in this study displayed good
performance in terms of sensitivity, stability,
resolution and peak density. This type of microchip
can also be used for detection of various other
organic or inorganic compounds, or can be
integrated with microfluidic modules on a µTAS.
REFERENCES
In-Je Yi, Ju-Ho Kim, Y. J. Choi, C. J. Kang, Yong-Sang
Kim, 2006. A disposable biosensor with Prussian blue
deposited electrode, Microelectronic Engineering, 83
(2006) 1594–1597.
Ju-Ho Kim, C. J. Kang, Yong-Sang Kim, 2005.
Development of a microfabricated disposable
microchip with a capillary electrophoresis and
integrated three-electrode electrochemical detection,
Biosensors and Bioelectronics 20 (2005) 2314–2317.
Gi-Sung Joo, Sandeep Kumar Jha, Yong-Sang Kim, 2009.
A capillary electrophoresis microchip for
amperometric detection of DNA, Current Applied
Physics 9 (2009) e222–e224.
Dolnik V., Liu S., Jovanovich S., 2000. Capillary
electrophoresis on microchip, Electrophoresis, 27, 41–
54.
Woolley A. T., Lao K., Glazer A. N., Mathies R. A., 1998.
Capillary Electrophoresis Chips with Integrated
Electrochemical Detection, Analytical Chemistry, 70,
684–688.
Jacobson S. C., Koutny L. B., Hergenroder R., Moore A.
W., Ramsey J. M., 1994. Effects of Injection Schemes
and Column Geometry on the Performance of
Microchip Electrophoresis Devices, Analytical
Chemistry, 66, 1107–1113.
Culbertson C. T., Jacobson S. C., Ramsey J. M., 2000.
Microchip Devices for High-Efficiency Separations,
Analytical Chemistry, 72, 5814–5819.
MICROCHIP CAPILLARY ELECTROPHORESIS DEVICE FOR AMPEROMETRIC DETECTION OF DNA WITH
REDOX INTERCALATION
287