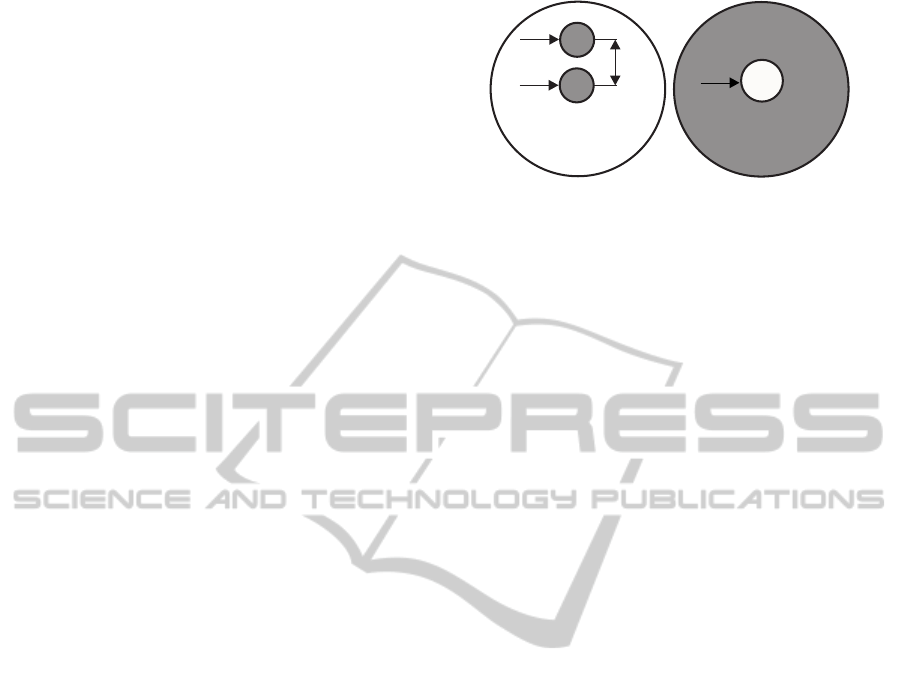
munication channels. The experimental equaliza-
tion of crosstalk within frequency non-selective op-
tical MIMO systems has attracted a lot of research
(Sch¨ollmann and Rosenkranz, 2007), (Sch¨ollmann
et al., 2008). By contrast, frequency selective
MIMO links require substantial further research,
where spatio-temporal vector coding (STVC) intro-
duced by RALEIGH for wireless MIMO channels
seems to be an appropriate candidate for optical trans-
mission channels too (Raleigh and Cioffi, 1998),
(Raleigh and Jones, 1999).
In this contribution the spatial multiplexing (SM)
is implemented at the transmitter side via different
sources launching light with different offsets into the
fibre. At the receiver side, i.e. at the fibre end, various
spatial filters are implemented (Pankow et al., 2011).
By launching light with different offsets into the fi-
bre, different mode groups are activated, which prop-
agate along the fibre with different speed and atten-
uation. Together, with the crosstalk between the dif-
ferent mode groups, the classical MIMO channel is
formed, where the most beneficial choice of the num-
ber of activated MIMO layers and the number of bits
per symbol offer a certain degree of design freedom,
which substantially affects the performance (Ahrens
and Benavente-Peces, 2009).
Against this background, the novel contribution
of this paper is that based on channel measurements
within a (2 × 2) optical MIMO system, the perspec-
tive of the MIMO philosophy within the field of op-
tical transmission systems is elaborated. Our results
show that even for relatively long (e. g. 1,4 km) trans-
mission lengths high data rates (e. g. 10,24 Gbps)
are feasible and that the choice of the number of bits
per symbol and the number of activated MIMO layers
substantially affects the performance of a MIMO sys-
tem, suggesting that not all MIMO layers have to be
activated in order to achieve the best BER.
The remaining part of this paper is organized as
follows: Section 2 introducesthe optical MIMO chan-
nel. The crosstalk impact within the optical MIMO
channel is studied in section 3, while the associated
performance results are presented and interpreted in
section 4. Finally, section 5 provides some conclud-
ing remarks.
2 OPTICAL MIMO CHANNEL
In order to comply with the demand on increas-
ing available data rates, systems with multiple in-
puts and multiple outputs, also called MIMO sys-
tems (multiple-input multiple-output), have become
indispensable and can be considered as an essential
10µm
10µm
TX
2
TX
1
ξ
RX
2
RX
2
RX
1
r
Figure 1: Forming the optical MIMO channel (left: light
launch positions at the transmitter side with a given eccen-
tricity ξ, right: spatial configuration at the receiver side as a
function of the mask radius r).
part of increasing both the achievable capacity and
integrity of future generations of communication sys-
tems (K¨uhn, 2006), (Zheng and Tse, 2003).
In this work the potential of the MIMO philoso-
phy in optical channels is elaborated, based on chan-
nel measurements. Basically, light launched at differ-
ent positions within the fibre activates different mode
groups, which propagate along the fibre with differ-
ent speed. Low order mode groups, activated by light
launched into the center of the fibre, lead to a power
radiation pattern concentrated at the center of the fiber
end whereas higher order modes, activated by light
launched at an off-center position, e. g., with a given
eccentricity ξ, within the fibre, lead to power radiation
pattern concentrated at the off-center of the fiber-end.
Therefore, by launching light into the fibre with given
eccentricities, as highlighted in Fig. 1, different spa-
tially separated power distribution pattern can be ob-
tained at the receiver side to form the optical MIMO
channel.
According to Fig. 1, the optical input TX
1
was ad-
justed to launch light into the center of the core (center
launch condition), whereas for the optical input TX
2
a given eccentricity ξ was chosen (off-center launch
condition). The activated modes can be separated at
the fibre end by the corresponding power distribu-
tion pattern. Fig. 2 illustrates the simulated power
distribution pattern by activating low- and high-order
modes. The simulations are in good agreement with
the measured power radiation pattern as depicted in
Fig. 3 for different parameters of the eccentricity ξ.
Now, spatial ring filters at the end of the transmission
line as depicted in Fig. 1 have been applied for chan-
nel separation. These spatial filters have been pro-
duced by depositing a metal layer at fiber end-faces
and subsequent ion milling (Pankow et al., 2011),
(Pankow et al., 2010).
Together, with the crosstalk between the different
mode groups, the classical MIMO channel is formed
(Fig. 4) (Pankow et al., 2011), (Pankow et al., 2010).
OPTICAL MIMO MULTIMODE FIBER LINKS - Channel Measurements and System Performance Analysis
129